Research Article
Endogenous archeological sciences: Physiology, Neuroscience, Biochemistry, Immunology, Pharmacology, Oncology and Genetics as instrument for a new field of investigation? Modern global aspects for a new discipline
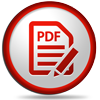
Luisetto M1*, Naseer Almukhtar2, Ghulam Rasool Mashori3, Ahmed Yesvi Rafa4, Farhan Ahmad Khan5, Gamal Abdul Hamid6, Luca Cabianca7 and Behzad Nili-Ahmadabadi8
1Applied Pharmacologist, European Specialist Lab Medicine, Independent Researcher, Italy
2Professor, Physiology, College of Medicine, University of Babylon, Hilla, Iraq
3Professor of Pharmacology, People University of Medical & Health Sciences for Women, Nawabshah, Pakistan
4Founder and President, Yugen Research Organization (YRO), Independent Researcher, Bangladesh
5Associate Professor and Head Dept. of Pharmacology, Govt. Medical College, Shahdol, Madhya Pradesh, India
6Professor, Hematology Oncology, University of Aden, Aden, Yemen
7Biomedical Laboratotry, Italy
8Nano Drug Delivery (A Product Development Firm), Chapel Hill NC, USA
*Address for Correspondence: Luisetto M, Applied Pharmacologist, European Specialist Lab Medicine, Independent Researcher, 29121, Italy, Email: [email protected]
Dates: Submitted: 27 November 2018; Approved: 13 December 2018; Published: 14 December 2018
How to cite this article: Luisetto M, Almukhtar N, Mashori GR, Rafa AY, Khan FA, et al. Endogenous archeological sciences: Physiology, Neuroscience, Biochemistry, Immunology, Pharmacology, Oncology and Genetics as instrument for a new field of investigation? Modern global aspects for a new discipline. J Neurosci Neurol Disord. 2018; 2: 065-097. DOI: 10.29328/journal.jnnd.1001017
Copyright License: © 2018 Luisetto M, et al. This is an open access article distributed under the Creative Commons Attribution License, which permits unrestricted use, distribution, and reproduction in any medium, provided the original work is properly cited.
Keywords: Inside; Endogenous; Archeology; Physiology; New scientific discipline; Archeo-toxicology; Archeo-oncology; Filogenetic; Ontogenetic; Pharmacological research
Abstract
In this work is analyzed scientific literature involved in human evolution to be used as an archeological Pathway to link different sciences in an overall new discipline.
A rational classification of single evidence make possible to better understand under new light some Physiological process.
The archeological instrument to be applied in other field like biology or other sciences.
Introduction
Today is interesting to submit to the scientific world a new field of investigation more structured that we can denominate Endogenous archeology.
A new field in which collect different research works from different discipline In order to correctly classify Endogenous process and structure of a sort of archeological provenience but today inside us.
In example is possible to verify physio-pathology of limbic system (amygdala) related relevant facts involving human living also in today time.
Archeology science not to be consider only related human product and man facts but also an inside disciple to verify archeological process related to mind- set kinetics and to other system or organs.
Brain, mind, immunologic system and other relevant physiological functions are deeply influenced by a primitive structure and to deeply understand the meaning of this complex system inside us make possible to better explain today Human behavior and physiology and other process.
To better explain this approach is possible to think in example the protection factor involved in auto-prevent poisoning form toxic plants or maternal instinct to protect fetus and other as sexual behavior, sleeping profile, fear and anger management, cooperative behavior et other strictly related whit evolutive Pathways.
Other facts can be considered to adequately set the endogenous archeo- structure:
The emato- encefalic barrier can be related to a ancestral world with higher toxic risk than the actual environment?
The same instinct is related to ancestral process (Figures 1-4).
lFigure 3: EEG of the patient: Periodic discharges and focal slowing in the left fronto-temporal areas, visible in an early stage of the HSV-1 encephalitis.
Why in human brain are present oppiaceus receptor strictly related to opiaceus alkaloids?
And why in some neurological disease are often present cellular inclusion like prot. TAU and similar?
Is possible verify that in hibernant mammalians reduction of metabolism make possible to adequate survive in real prohibitive condition.
And related Neurotransmitter and receptorial status: this can be related to ancestral needs?
(Sympatic system so named by GALENO (130-200 DC) to describe nerves functions as link with periferical organs. A synaptic coordination between various parts of the body, Prasimpatic: near to sympatic system, and other receptorial system like dopaminergic, seroto ninergic, purinergic, oppiacei receptors and so on)
Why insulin human production follow a circadian profile related the food level of intake?
Why bowel contract itself like ancient warms?
And related Maslow’s hierarchy of needs why in the basis of pyramids are present physiological need?
And related atetosic – tentacular movement in HUNTINGTON COREA disease? Inaccurate movements and in coordinated and its similarity with some aquatic invertebrate (see nervous system of this last compared to human).
Other examples can be : the atrofized men breast, human coccige, peach fuzz in al body, and other vestigial organs related to evolutionism like Ciecal appendix, ancestral erbivor residual bowel, navel, timus ant its immunologic profile.
Vomero nasal organ, now out of encephalon, in past function in detect other individuals ferormons
Darwin lobuli: residual structure by which animals can move and better orienting auricolar pavilions.
L-gulonolattone oxidase pseudo- gene: present in superior anuimals but in human not codify for no one gene.
The human Taurodontic teeth related the actual dietary behavior, not necessary a great functional differentiation between teeth (canins).
And Observing also the different in bipedal and in quadrupedal primates; the monkeys show the same rate in urinary infections like actual humans?
They need the same amount of antimicrobials / year due by UTI infectious disease? And why?
Verifying the evolution and final status of the lower urinary tract position related to gravity is possible to verify the best condition to have maximum urinary flow also in actual bipedal humans.
The human osmotic blood pressure related to NACL concentration remember an evolution from sea.
Other examples can be the Vomit protective reflex, and related genetic profile:
Human and other organism Junk DNA not codifying
Very interesting is also the Brain evolution: palleocortex (maintain the olfactory function), archicortex (and its affectivity control in first mammalians), neocortex related also to cognitive process.
The Primitive brain (reptilian brain or spinal bubble) is involved in involuntary movement, heart rate, breath et other, the intermediate brain or limbic system involved in emotion like fear anger, sensitive and motor response (first mammalian) and neocortex associative (associated cognitive and relational functions) show a n evolution of structure associate to a more complex functions.
The Unicellular or pluricellular and then in Vertebrates evolution in motor systems (in fish, reptile, birds, mammalians) show a common evaluative way.
Ancient Fish presented limited motor function related to the see environment (under a spinal cord control and pallidus nucleus) in an ondulatory movement involving full body.
Reptile and anfibius developed other motor abilities related the new environment out of sea: new and complex motor need in the shift from sea to paludes and ground the Development of Striatus nucleus in reptile (involved in crawl movement), as well as an high development of cerebellum in birds, improved movement of the body extremities and not only of the full body like in fish and reptile.
Other High sensorial organ in birds as instrument to facilitate flying, Birds take care of progeny, lives in family groups (on contrary of reptile) present higher and new instinct.
In evolution in mammalians increased the encephalic control and reduced the automatism.
See in example progression movement in child (6 month -1 year): oscillate like fish, slither like reptile, crawl like mammalian, climb up and the walk.
In mammalians evolution 90% of neurocortex is involved in associative role (10% sensitive and motor)
In The gradual evolution of vertebrates with adding of new sovrastructure in brain the more recent level not needed other conduction ways to connect central nervous systems to muscle.
In striatus polineuronic way present a slow process and so in neurocortex a new direct way named pyramidal (new system named pyramidal added to the old system named extrapitramidal).
Pyramidal system make possible more precision in movement and rapidity in muscle response.
In mammalians tele- encefalization reduced the spine cord control in relation to in birds (in this last higher motor automatism).
And in other animals is possible to see related evolutive process:
in cetaceus: bone draft posterior limb.
Wings in not flying birds, Pelvic belt in snake
Rudimental eyes in Amblyopsidae fish
Notocord vertebrate evolution and metameric organization
Gill evolution et other
Material and Methods
With an observational approach some relevant literature (from PUBMED database) are analysed in order to produce a final conclusion and to produce an Researchgate PROJECT dedicated opened to many researcher from different field like : neuroscientist, biologist, archeologist, psychologist, genetics, immunologist anatomist, physiologist and many other.
Results
From scientific literature is possible to observe
In article: Amygdala pharmacology and crime behavior, dysfunctions to be considered as a disease: “neuro-pharmacology research of amygdala can give the response also using imaging Techniques. Studies demonstrated that Amygdala activation is involved in aggressive behavior” [1].
And In publication Mindset kinetics and some depression status: A new quantitative model under biochemical - toxicology approach? 2018 is reported: “According Pubmed Health database: Depression is a state of low mood and aversion to activity that can affect a person’s thoughts, behavior, feelings and sense of well-being (moderate or severe).
Can we consider some depression status due to high amount of stress ant stimulus? Or continuous stress in a limited (or long) period? How can react mindset and brain in management a high amount of negative stressing thinking? Observing relevant literature also mindset kinetics must be considered to better classify this kind of disorder under a specific endogenous –exogenous biochemical-toxicological aspect.
Concept as Kinetics, reaction velocity limits, saturation of the systems, residual buffer properties are currently used in biochemistry and related discipline.
This concepts can be applied also in some depression condition to better explain some biological phenomena? [2].
And in article Brain and Transmission Signal Modulation 2017: “In activation of amygdala the response is more rapid than when involved the frontal cortex” [3].
Carey HV et al. writed, “Mammalian hibernators undergo a remarkable phenotypic switch: it involves great changes in physiology, morphology, and behavior in response to periods of unfavorable environmental conditions. The ability to hibernate is found throughout the class Mammalia and appears to involve differential expression of genes common to all kind of mammals, rather than the induction of novel gene products unique to the hibernating state. The hibernation season is characterized by extended bouts of torpor, minimal body temperature (Tb) can fall as low as -2.9 degrees C and metabolism can be reduced to 1% of euthermic rates. Many biochemical - physiological processes exploit low temperatures to lower reaction rates but retain the ability to resume full activity upon rewarming. Other critical functions must continue at physiologically relevant levels during torpor and be precisely regulated even at Tb values near 0 degrees C. Research using new instruments as molecular and cellular biology is beginning to reveal how hibernators survive repeated cycles of torpor and arousal during the hibernation season. Genomic and proteomic technologies are needed to further better define the differentially expressed genes that distinguish the summer euthermic from winter hibernating states. Detailed understanding of hibernation from the molecular to organismal levels should enable the translation of this information to the development of a variety of hypothermic - hypometabolic strategies to improve outcomes for human and animal health” [6].
According Davidson I, “archeology of cognition is concerned primarily with the evolutionary emergence of the cognition particular to modern humans but there is an implication for the evolution of cognition among modern humans. Archeological evidence can provide important insights into the evolutionary emergence of human cognition, but theoretical considerations are fundamental in understanding what sorts of cognition there might have been between the ape-like common ancestor and modern humans. Archeology is the only source of evidence for the behavior associated with such theoretical stages. Cognitive archeology, therefore, involves an iterative interaction between theory from outside archeology and more or less direct evidence from the past. This review considers the range of possible evidence from archeology and genetics and summarizes the results of analysis of non-human primates particularly to assess characteristics of the last common ancestor (LCA) of apes and humans. The history of changes in size and shape of the brain since separation from other apes introduces the need to assess the appropriate cognitive theories to interpret such evidence. The review concentrates on two such approaches: Baddeley’s working memory model as interpreted by Coolidge and Wynn, and Barnard’s interacting cognitive subsystems as it has been elaborated to define the cognitive conditions for hominins between the LCA and modern people. Most of the rest of the review considers how the evidence from stone tools might be consistent with such theoretical models of cognition. This evidence is consistent with views that modern human behavior only emerged in the last 100,000 years (or so) but it gives an explanation for that in terms of cognition” [7].
Simon Neubauer et al. showed that, “Modern humans have large - globular brains that distinguish them from their extinct Homo relatives. The characteristic globularity develops during a prenatal / early postnatal period of rapid brain growth critical for neural wiring and cognitive development. However, it remains unknown when and how brain globularity evolved and how it relates to evolutionary brain size increase. On the basis of Tc scans and geometric morphometric analyses, we analyzed endocranial casts of Homo sapiens fossils (N = 20) from different time periods. Our scientific data show that, about 300,000 years ago, brain size in early Homo. Sapiens already fell within the range of present day humans. Brain shape, evolved gradually within the H. sapiens lineage, reaching present-day human variation between about 100,000 - 35,000 years ago. This process started after other key features of craniofacial morphology appeared modern and paralleled the emergence of behavioral modernity as seen from the archeological record. Our findings are consistent with an important genetic changes affecting early brain development within the H. sapiens lineage since the origin of the species and before the transition to the Later Stone Age and Upper Paleolithic that mark a full behavioral modernity” [8].
Bonett RM, “Analyzing variation in rates of evolution can provide an important insights into the factors that constrain trait evolution, as well as those that promote diversification. Metazoan endocrine systems exhibit an apparent variation in evolutionary rates of their constituent components at multiple levels, yet few studies have quantified these patterns and analyzed them in a phylogenetic context. This may be in part due to historical and current data limitations for many endocrine components and taxonomic groups. Recent technological -advancements such as high throughput sequencing technique provide the opportunity to collect large-scale comparative data sets for even non-model species that will produce a fertile data landscape for evolutionary analyses of nucleic acid and amino acid based endocrine components. I summarize evolutionary rate analyses that can be applied to categorical and continuous endocrine traits, and also those for nucleic acid and protein-based components. I emphasize the analyses that could be used to test whether other variables (ecology, ontogenetic timing of expression.) are related to the patterns of rate variation and endocrine component diversification. The application of phylogenetic based rate analyses to the comparative endocrine data will greatly enhance our understanding of the factors that have shaped endocrine system evolution” [9].
Charles L Nunn et al., “Sleep is essential to cognitive function and health in humans, yet the ultimate reasons for sleep—i.e. ‘why’ sleep evolved—remain mysterious. We integrate the findings from human sleep studies, the ethnographic record, and the ecology and evolution of mammalian sleep to better understand sleep along the human lineage and in the modern world. Compared to the other primates, sleep in great apes has undergone substantial evolutionary change, with all great apes building a sleeping platform or ‘nest’. Further evolutionary change characterizes human sleep, with humans having the shortest sleep duration, yet the highest proportion of rapid eye movement sleep among the primates. These changes likely reflect that our ancestors experienced fitness- benefits from being active for a greater part of the 24 h cycle than other primates, potentially related to advantages arising from learning, socializing and defending against predators and hostile conspecifics. Perspectives from the evolutionary medicine have implications for understanding sleep disorders; we consider these perspectives in the context of: insomnia, narcolepsy, seasonal affective- disorder, circadian rhythm disorders and sleep apnea. We also identify how the human sleep today differs from sleep through most of human evolution, the implications of these changes for global health and health disparities. Our review highlights importance of the phylogenetic comparisons in understanding human health, including well-known links between sleep, cognitive performance and health” [10].
Müller M, “Parasitic amitochondriate protists, representatives of early branches of the eukaryote evolution, differ considerably in their central, energy metabolism from mitochondrion-bearing cells. Differences are: significant metabolic functions of inorganic pyrophosphate, major role of iron-sulfur proteins in key metabolic steps and in hydrogenosome-bearing organisms the disposal of electrons by H2 formation. Cytochrome mediated electron- transport and electron transport-linked phosphorylation are absent. All the proteins which have been sequenced so far were found to be homologous to isofunctional proteins from other organisms. few reactions are catabolized by the proteins which are not homologous to enzymes performing similar reactions in other eukaryotes. Two significative different types of metabolism of amitochondriate protists can be distinguished: (1) without compartmentation and (2) with cytosol/hydrogenosome compartmentation. These metabolic- types have conserved certain traits present in ancestral eukaryotes before mitochondria became established [11].
Marco Fondi et al., “It is assumed that primordial cells had small genomes with simple genes coding for enzymes able to react with a wide range of chemically related substrates, interconnecting different metabolic routes. New kind of genes coding for enzymes with a narrowed substrate specificity arose by paralogous duplications of ancestral ones and evolutionary divergence. So new metabolic pathways were built up by primordial cells. Useful hints to disclose the origin / evolution of ancestral metabolic routes and their interconnections can be obtained by comparing sequences of enzymes involved in the same or different metabolic routes.
The lysine, arginine, and leucine biosynthetic routes represent very interesting study-models. Some of the lys, arg, leu genes are paralogs; this led to the suggestion that their ancestor genes might interconnect the three pathways. The aim of this work was to trace the evolutionary pathway leading to the appearance of the extant biosynthetic routes and to try to disclose the interrelationships existing between them and other pathways in the early stages of the cellular evolution” [12].
According Dariush D Farhud et al., “The evolution of human blood groups, without doubt, has a history as old as man himself. There are at least 3 hypotheses about the emergence and mutation of human blood groups. The Global distribution -pattern of blood groups depends on various environmental factors, such as disease, climate, altitude, humidity. In this study, the collection of main blood groups ABO and Rh, along with some minor groups, are presented. Various investigations of blood groups from Iran, particularly a large sampling on 291857 individuals from Iran, including the main blood groups ABO and Rh, as well as minor blood groups such as Duffy, Lutheran, Kell, KP, Kidd, and Xg, have been reviewed.
It was not until 1900, when Karl Landsteiner at the Vienna University, discovered why some blood transfusions were successful while others could be deadly. Landsteiner discovered the ABO blood- group system by mixing the red cells and serum of each of his staff. He demonstrated that: the serum of some people agglutinated the red cells of others. From these first experiments, he identified three types, called A, B and C (C was later to be re-named O for the German “Ohne”, meaning “without”, or “Zero”, “null” in English). The fourth less frequent blood group AB, was discovered one year later, Landsteiner received the Nobel Prize in physiology and medicine for his work.
The gene that determines human ABO blood type is located on the chromosome 9 (9q34.1) and is called ABO glycosyl-transferase. The ABO locus has three main allelic forms: A, B, and O, as mentioned above and each of them is responsible for production of its glycoprotein. The combination of alleles that are inherited from parents that determines which glycoproteins (antigens) are found on persons’ blood cells and thereby their ABO blood type.
Genesis and Evolution: investigations have demonstrated on monkeys, human blood groups are very old genetic indicators which have evolved during a several million years. Based on the primary races hypothesis, it was thought that in the three major races of man, blood groups A in Europe, B in Asian, finally O in South America have been emerged and gradually due to the migration and mixing of the races, became the present situation. But we know that in each continent, the isolated populations are seen that have completely different blood groups. In example, there is relatively high prevalence of blood group O in Siberian inhabitants; also this blood group is very common in some parts of Switzerland.
Related to this theory, the old races have O blood group, such as Red Indians of South America, and Eskimos that among them the frequency of O blood group is between about 75–100%. In most of recent ethnic- groups A and B blood groups are dominant.
In other hypothesis, the first blood group had been AB blood group, which gradually and over the time due to genetic mutations was resulted in A and B and finally O blood groups. Base on this theory, few million years ago all people have had type O blood only, which is more resistant against many infectious diseases. After discovery of the first human blood groups (ABO) by Karl Landsteiner in 1901, gradually from 1927, other blood groups were also discovered and reported which its collection is given in. It is important to mention that Landsteiner together with his American colleague Alexander Wiener discovered the Rh blood group and reported it in 1940, 1941. K. Landsteiner was born on 14th June 1868, in Vienna. Landsteiner in his 17th scientific paper in 1901 reported blood group ABO which was displayed at the beginning with letters ABC. In 1930, he received the Nobel Prize for this discovery.
In addition to the known blood groups, nearly twenty public antigens and also sixty-specific antigen or family antigen (Private Ag) have been reported.
Moreover, the main blood groups ABO, gradually discovered -reported which the most notably of them are as:
A subgroups, including A1, A2, A3, and also rare types A4, A5, A6, Z, X, End, boutu, g, i.
B subgroups, including B1, B2, B3, the rare types w, x, v, m.
Subgroups, including O1, O2, O3, other types such as Yy, Hh, Xx, and Bombay” [13].
According de Boer B, “Speech is the physical signal used to convey spoken language. Because of its nature, speech is both easier to compare with other species’ behaviors and easier to study in the fossil record than other aspects of language. I argue that the convergent fossil evidence indicates adaptations for complex- vocalizations at least as early as the common ancestor of Neanderthals and modern humans. I argue that it is unlikely that language evolved separately from speech, but rather that gesture, speech, and song coevolved to provide both a multimodal communication system and a musical system. Coevolution also have played a role by allowing both the cognitive and anatomical adaptations to language and speech to evolve in parallel. Although such a coevolutionary scenario is complex, it is entirely plausible from a biological point of view” [14].
Cecilia Heyes, “Humans are animals that specialize in thinking / knowing, and extraordinary cognitive abilities have transformed every aspect of our lives. In contrast to our chimpanzee cousins and Stone Age ancestors, we are complex political/ economic,/scientific / artistic creatures, living in a vast range of habitats, many of which are our own creation. Studies on human cognition evolution asks what types of thinking make us such peculiar animals, and how they have been generated by evolutionary processes. New research i looks deeper into the evolutionary history of human cognition, and adopts a more multi-disciplinary approach than earlier Evolutionary Psychology. It is informed by comparisons between humans and a range of primate / non-primate species, and integrates findings from anthropology, archaeology, economics, evolutionary biology/neuro-science/ philosophy / psychology. Using this methods, recent research reveals profound commonalities, as well striking differences, between the human and non-human minds, and suggests that the evolution of human cognition has been much more gradual and incremental than previously assumed. It accords a crucial roles to cultural evolution, techno-social co-evolution and gene culture co-evolution. These have produced domain-general developmental processes with extraordinary power that makes human cognition, and human lives, unique.
Chimpanzees lead a quite interesting lives. They build nests, they form alliances with other members of their troop and use simple tools, sticks to fish for termites and stones to open nuts. We are reminded by a comedic images of chimpanzees wearing clothes, using computers, the lives of our closest evolutionary relatives are very different from our own. Translated as: wise man, Homo sapiens is an optimistic sobriquet; translated as: knowing man it is merely descriptive of our species. We are animals that specialize in thinking and knowing—in cognition— our extraordinary cognitive powers have enabled us to do remarkable things. We have transformed our eating habits with agriculture and cooking, and transformed our habitats with buildings, bridges and roads. Compared to our chimpanzee cousins, we can travel over vast distances, moving our whole bodies in cars, planes and space craft, and moving our minds to yet more remote places with radio telescopes and electron microscopes. We are political /economic animals, negotiating agreements that affect millions of people, and trading instantly in rarefied currencies with completely anonymous strangers in different time zones. We know about time, we understand it to some degree and we can measure it precisely. We communicate with the symbol/spoken and written languages—and using these languages, we have developed extensive knowledge of our own history and diversity, and about all aspects of the natural and physical worlds. Our lives are enriched by a fabulous beautiful, intricate and provocative objects—by art, architecture, music, dance—and, in addition to developing the weaponry of ‘shock and awe’, we engage in sports, complex rituals that channel and redirect the impulse to fight.
How has evolution produced some creatures with minds capable of these remarkable feats? They represent new thinking; leading edge, evidence-based theory about the new forms of cognition that emerged in the course of human evolution. This new theory and evidence come from a range of disciplines, including anthropology, archaeology, economics, evolutionary biology, neuroscience, philosophy and psychology. The new forms of cognition include causal reasoning,/imitation,language/metacognition and theory of mind.
Over the past 25 years, studies on the evolution of human cognition has been dominated by a type of evolutionary psychology promoted most prominently by Cosmides and Tooby. This framework, which I will identify using initial capitals (‘Evolutionary Psychology’). It suggests that the human mind consists in a large collection of computationally distinct ‘modules’. Each modules is a way of thinking that was shaped by natural selection to solve a particular type of problem faced by our Stone Age ancestors—for example, communicating, prey stalking, disease avoidance, mate choice and coalition formation. Evolutionary Psychology’s central metaphor is the Swiss- Army knife. It casts the evolved human mind as a set of cognitive gadgets, each specialized to learn, remember and reason about particular types of information. It has drawn attention to the need to integrate cognitive science with the evolutionary biology in order to explain not only how brains and behavior have evolved, but also the evolution of the ‘middle man’—the cognitive processes, often characterized as computational software, which are instantiated by the brain and control behavior. This new thinking that runs through this theme issue, sees the human mind as more like a hand than a Swiss Army knife. The hand is a multi-purpose instrument of a very different kind to the Swiss Army knife. It has a deep evolutionary history, rooted in the earliest emergence of the pentadactyl limb, and incorporates many genetic adaptations. The human hand is capable of performing a wide / open-ended variety of technical and social functions. It can strip the defensive spines from a piece of fruit, making it safe to eat, in Thai dancing it can also signal the smallest nuances of emotion. The human hand performs with equal facility a vast array of tasks that natural selection did and did not ‘foresee’.
This work introduces the theme issue by contrasting our ‘new thinking’ with Evolutionary Psychology in relation to three closely related questions about the evolution of human cognition: When did the most important changes take place? How did the changes happened? What have the changes produced? “[15].
According paper Evolutionary change in agriculture: the past, present and future in 2010 reported that, “we draw together a series of diverse studies that provide a sample of some of the ways in which evolution driven by both conscious / unconscious selection by humans has shaped the development of modern agriculture. Agriculture has been a crucible of evolutionary change ever since its inception thousands of years ago, and this change permeates agricultural endeavors at all levels of biological organization, ranging from the individual gene through to whole communities. Agro-ecosystems thus provide one most cogent examples of situations where anthropogenic effects are major determinants of biotic interactions within and among species and communities, suggesting a central role for the application of evolutionary principles. This is particularly the case, given global concerns regarding food production / food security, and increasingly, the expectation that agricultural- productivity gains must be achieved with greater efficiencies, and reduced environmental impact.
In the development of the human society, the shift to a relatively settled way of life from a nomadic hunter-gatherer life-style was enormously profound, and made possible only by the development and adoption of early agricultural practices. This change in human life-style had major impacts on the environment of agriculture, hence the traits and characteristics of plants and animals that were favored both consciously and unconsciously by humans. Very often traits suited to species growing without human protection/husbandry were not suitable for agriculture and were rapidly lost (seed shattering), while others were specifically favored by the more protected environment agriculture provided, and increased in frequency (seed retention).
As agriculture developed, the environment of the field and paddock became increasingly differentiated from that of the natural environments in which plants and animals originally evolved. For the plants, nutrient availability generally rose, plant density and genetic uniformity increased, and the balance in competition shifted from inter-specific considerations to intra-specific ones (particularly with general reduction in species diversity). Tilling and crop rotation were further agronomic practices that were introduced partly for fertility reasons but also for control of natural enemies, as rotation causes shifts in whole fungal pathogen communities. Another generation of changes to tilling practices – minimum tillage – is to some extent now altering ecological, evolutionary interactions at the plant–soil interface towards a different set of fungal control issues. For the animals, domestication created a more predictable environment with increased resource availability during harsh times and protection from predators, but increased threats from contagious diseases, all subtly influencing the evolutionary make-up of our livestock.
In last few thousand years, domestication, selection and hybridization, both unconscious and conscious, has also led to significant changes in the appearance of plants and animals and their nutritional value.
Example are seen in virtually all plant and animal species that are farmed. In horticulture, this diversity is highly prized in the form of different varieties that are preserved for subtle variations in flavor, texture or simply appearance (e.g. in potato, tomato, apple). In a similar way, extensive selection in farmyard fowls (chickens, ducks, geese and turkeys), and in pigs, sheep and cattle have given rise to very many distinctive breeds that differ in milk production, flesh texture and flavor, and obvious appearance, as well as in less obvious traits, such as patterns of social behavior. Extensive agriculture has also seen similar major changes that have resulted in significant increases in yield / productivity. In plants one of the most dramatic changes to have occurred within a species is found in the emergence of modern high-yielding hybrid maize from its close relative teosinte, and the subsequent application of a number of induced mutations and the introduction of an F1 hybrid system. Similarly dramatic changes have occurred in meat and fibre production and quality in selected beef and sheep varieties respectively. In the history of agriculture, changes in agronomic and animal husbandry practices and in the crops and animals being farmed have had collateral effects that have changed the balance and intensity of different selective forces. This has been particularly apparent in the plants where increasing nutrient status (particularly the nitrogen) generally favors the growth and development of insect and fungal pests – the numbers of which are frequently then further exacerbated by increased plant density (this increases humidity and thus conditions for fungal spore germination; and reduces inoculum loss during transmission) and genetic uniformity of crops. As selection by humans shifted to a conscious understanding of genetics, the focus also increasingly centred on the accumulating single major gene traits because these could be more readily manipulated.”(16).
F Luca et al. write that, “Through cultural innovation and changes in habitat and ecology, there have been a number of major dietary shifts in human evolution, including meat eating, cooking, and those associated with plant and animal domestication. The identification of signatures of adaptations to such dietary changes in the genome of extant primates humans included may shed light not only on the evolutionary history of our species, also on the mechanisms that underlie common metabolic diseases in modern human populations. The evolutionary history of hominins has been characterized by significant dietary changes, which include introduction of meat eating, cooking, and the changes associated with plant and animal domestication. Decades of anthropological research studies have been devoted to elucidating this dietary history, in part because these shifts were likely associated with major anatomical and cultural changes (the increase in relative brain size and the advent of modern civilization via agriculture).This reconstruction is crucial for understanding the evolutionary context of our modern diets and the diseases often associated with them.
Related to the historical reconstruction of hominin diets, molecular evolutionary analyses have been used to interrogate the genome for signals of genetic adaptations to different dietary regimes. A major advantage of many evolutionary genetic approaches is that they do not necessarily require strong assumptions about the specific genes and alleles that were targets of diet-related selective pressures. Evolutionary genetic analyses have the potential not only to inform existing adaptive hypotheses of hominin dietary history, to help generate new ones.
We bring together these 2 areas of inquiry, namely anthropology and evolutionary genetics, to highlight their recent findings related to human dietary history and to discuss the limitations of different approaches. We start by providing a brief overview of the major dietary shifts in hominin -evolution and discussing evolutionary genetics methods and approaches used to identify signals of natural selection. We then review the results of genetic studies aimed at detecting the loci that played a major role in dietary adaptations” [17].
Tattersall write that, “Human beings are distinguished from other organisms by their symbolic way of processing information about the world. This unique cognitive style is qualitatively different from all the earlier hominid cognitive styles, and is not simply an improved version of them. The hominid fossil / archaeological records show clearly that biological and technological innovations have typically been highly sporadic, and totally out of phase, since the invention of stone tools some 2,5 million years ago. They a confirm that this pattern applied in the arrival of modern cognition: the anatomically recognizable species Homo sapiens was well established long before any population of it began to show indications of behaving symbolically. This places the origin of symbolic thought in the realms of exaptation, whereby new structures come into existence before being recruited to new uses, and of emergence, entire new levels of complexity are achieved through new combinations of attributes unremarkable in themselves. These phenomena involve entirely routine evolutionary -processes; special as we human beings may consider ourselves, there was nothing special about the way we came into existence. Modern human cognition is a very recent acquisition; and its emergence ushered in an entirely new pattern of technological (and behavioral) innovation, in which constant change results from the ceaseless exploration of the potential inherent in our new capacity” [18].
According Bar-Rogovsky H et al. showed that, “Serum para-oxonases (PONs) are detoxifying lactonases that were first identified in mammals. 3 mammalian families are known: PON1,2, and 3 that reside primarily in liver. They catalyze the same reaction, lactone hydrolysis, but differ in their substrate specificity. Some are highly specific, others have a broad specificity profile. The evolutionary origins and substrate specificities of PONs therefore remain poorly understood. Here, we report a newly identified family of bacterial PONs, and the reconstruction of the ancestor of the three families of mammalian PONs. Both the mammalian ancestor and the characterized bacterial PONX_OCCAL were found to efficiently hydrolyze N-acyl homoserine lactones that mediate quorum sensing in many bacteria, including pathogenic ones. The mammalian PONs may therefore relate to a newly identified- family of bacterial, PON-like “quorum-quenching” lactonases. The appearance of PONs in metazoa is likely to relate to innate immunity rather than detoxification. Unlike the bacterial PON, the mammalian ancestor also hydrolyzes, with a low efficiency, lactones other than the homoserine lactones, thus preceding the detoxifying functions that diverged later in two of the three mammalian families. The bi-functionality of the mammalian ancestor and the trade-off between the quorum-quenching and detoxifying lactonase activities explain the broad and overlapping specificities of some mammalian PONs vs the singular specificity of others” [19].
Gehring WJ, “the evolution of vision is retraced from its putative origins in cyanobacteria to humans. Circadian oscillatory clocks, phototropism, and phototaxis require the capability to detect light. Photosensory proteins allow us to reconstruct molecular phylogenetic trees. The evolution of the animal eyes leading from an ancestral prototype to the highly complex image forming eyes can be deciphered on the basis of evolutionary developmental genetic experiments and comparative genomics. As all bilaterian animals share the same master control gene, Pax6, and same retinal and pigment cell determination genes, we conclude that different eye-types originated monophyletically and subsequently diversified by divergent, parallel, or convergent evolution” (20).
Yi-An Ko et al., “Epigenetics refers to the functionally relevant modifications of the genome that do not involve a change in the nucleotide sequence. Examples of such modifications are DNA methylation and histone modifications. Both this modifications serve to regulate gene expression without altering the underlying DNA sequence. The epigenome encodes critical information to regulate the gene expression. The cellular epigenome is established during development and differentiation and maintained during cell division. These instructions are different in each cell type; therefore the epigenome is cell type specific. Nutrient availability and other environmental factors cause changes in the epigenome. Recent studies suggests the critical contribution of the epigenome to the development of complex gene-environmental diseases including chronic kidney diseases.
Epigenetics, meaning above (epi-) genetics, is the study field of gene expression regulation that cannot be directly attributed to changes in the DNA sequence. Among the 3 billion nucleotides in our genome, less than 2% are responsible for coding proteins. The rest of the sequences were long thought to be non-functional (‘junk’), possibly only there as a buffering zone for mutations. Recent research, especially those published by the ENCODE consortium, indicate that, by describing and annotating the cellular epigenomes, functional roles could possibly be assigned to as much as 80% of the genome. It seems that the instruction manual that governs gene expression is hidden somewhere in the ‘junk DNA’.
The epigenome involves ‘marking’ (i.e. chemical modification) of DNA or the associated proteins. Epigenetic marks include cytosine modification (mainly methylation) and histone tail modification. The epigenome is inherited during cell division to maintain cell identity. However, nutrition and other environmental factors change these chemical tags. These chemical tags (the epigenome) serve as the intersection between the stably inherited genome and the changing environment. Characterization of the epigenome has helped tremendously to define cell-type specific gene expression. A typical transcription unit in a multicellular eukaryote contains both clusters of proximal promoter elements and five types of cis-acting regulatory sequences (insulators, promoters, enhancers, silencers, and locus control regions). Insulator areas border and separate transcriptional units. Promoters are located at the 5′ end of transcription start site and contain elements called TATA box, which is bound by either a TATA-binding protein or a cluster of cytosine-guanine nucleotide pairs within the linear sequence, known as CpG islands (CGI). For target gene transcription to take place, not only promoters but also long- and short-range regulatory regions are needed. These cis-type gene regulatory regions are highly cell- type specific and are critical for cell-type specific transcription. Simultaneous binding of the transcription- factors to each other and to the long-and short-range regulatory regions, results in genomic DNA loops that join distant regulatory DNA sequences together” [21].
Joseph E Le Doux, “Basic tendencies to detect / respond to significant events are present in the simplest single cell organisms, and persist throughout all invertebrates and vertebrates. Within vertebrates, the overall brain plan is highly conserved, though differences in size / complexity also exist. The forebrain differs the most between mammals and other vertebrates. The classic notion that the evolution of mammals led to radical changes such that new forebrain structures (limbic system and neo-cortex) were added has not held up, nor has the idea that so-called limbic areas are primarily involved in emotion. Modern efforts have focused on the specific emotion systems, like the fear or defense system, rather than on the search for a general purpose emotion systems. Such research have found that fear circuits are conserved in mammals, including humans. Animal work has been especially successful in determining how the brain detects and responds to danger. Caution should be exercised when attempting to discuss other aspects of emotion, namely subjective feelings, in animals since there are no scientific ways of verifying and measuring such states except in humans.
The topic of emotion and evolution typically brings to mind Darwin’s classic treatise, Emotions in Man and Animals (Darwin- 1872). In this book Darwin sought to extend his theory of natural selection beyond the evolution of physical structures and into the domain of mind and behavior by exploring how emotions too might have evolved. Particularly important to his kind of argument was the fact that certain emotions are expressed similarly in people around the world, including in isolated areas where there had been little contact with outside world and thus little opportunity for emotional expressions to have been learned and culturally transmitted. This suggested to him that there must be a strong heritable component to emotions in people. Also important was his observation that certain emotions are expressed similarly across the species, especially closely related species, further suggesting that these emotions are phylo-genetically conserved.
With the rise of experimental brain research studies in the late 19 th century, emotion was one of the key topics that early neuroscientists sought to relate to the brain The assumption was that the emotion circuits are conserved across mammalian species, and that it should be possible to understand human emotions by exploring emotional mechanisms in the non-human mammalian brain.
I will first briefly survey the history of ideas about emotional brain, and especially ideas that have attempted to explain the emotional brain in terms of evolutionary principles. This will lead to a discussion of fear, since this is the emotion that has been studied most thoroughly in terms of brain mechanisms. The chapter will conclude with a reconsideration of what the term emotion refers to, and specifically which aspects of the emotion can be studied in animals and which must be studied in humans.
A Brief History of the Emotional Brain: The Rise and fall of the Limbic System Theory
All organisms, even single cell organisms, must have capacity to detect and respond to significant stimuli in order to survive. Bacteria, for example, approach nutrients and avoid harmful chemicals. With the evolution of multicellular, metazoan organisms with specialized systems, particularly a nervous system, the ability to detect and respond to significant events increases in sophistication.
Invertebrates, the oldest and largest group of multicellular organisms, exhibit a wide variety of types of nervous systems. However, all vertebrates share a common basic brain plan consisting of three broad zones (hindbrain, midbrain, forebrain) with conserved basic circuits. Differences in size and complexity exist. For example, the forebrain differs the most between mammals and reptiles. On the basis of such differences, the classic view of forebrain evolution emerged in the first half of the 20th century. According to this view, with the emergence of mammals, the forebrain plan underwent radical changes in which new structures, especially cortical structures, were added. These were layered over and covered the reptilian forebrain, which mainly consisted of the basal ganglia. First came “primitive” cortical regions in the early mammals. In these organisms the basic survival functions related to feeding, defense and procreation were taken care of by fairly undifferentiated (weakly laminated) cortical regions (primitive cortex, including the hippocampus and cingulate cortex) and the related subcortical areas (such as the amygdala) that were closely tied to the olfactory system. Later mammals added highly novel, laminated cortical regions (neocortex) that made possible enhanced non-olfactory sensory processing and cognitive functions (including learning and memory, reasoning, and planning capacities, and, in humans, language).
The principle that equated cognition with evolutionarily new cortex (neocortex) and emotion with older cortex- related subcortical forebrain regions culminated in Paul MacLean’s limbic system theory of emotion the term limbic was first used by the French anatomist Paul Broca as a structural designation for a rim of cortex in the medial wall of the hemisphere. Broca called this rim the limbic lobe (le grande lobe limbique) (limbic is from the Latin word for rim, limbus). MacLean built on the classic findings of comparative anatomists such as Herrick and Papez, and experimental findings from Walter Cannon, Phillip Bard and Henrich Kluver and Paul Bucy to transform the limbic lobe into an emotion system, the limbic system. The limbic syst. was defined anatomically as the primitive medial cortical areas and interconnected subcortical nuclei (including the amygdala and septum).
MacLean called the limbic system the paleo-mammalian brain (since it was said to have emerged with the evolution of early mammals), and contrasted it with the reptilian brain (basal ganglia and brainstem). In more recent mammals the neocortex, also called the neo-mammalian brain, was said by MacLean to increases in size and complexity at the expense of the limbic system. The decrease of the limbic system reduced the dependence of humans on base emotions, and the increase in the neo-cortex allowed humans greater control over remaining emotional circuits as well as greater cognitive capacities.
The limbic system concept stimulated much research in the 1950s- 70s. it has been criticized on a number of grounds, and has been rejected by many scientists. Because the limbic system concept continues to be referred to in some scientific circles and persists in many lay accounts of the brain, it is worth considering why it is not acceptable.
The theory presumes that the neo-cortex and limbic system are unique mammalian specializations. Neither of these ideas is accepted by contemporary comparative neuro-anatomists. Birds and reptiles, for example, have been shown to have structures that correspond with both mammalian neo-cortex and with MacLean’s cortical and subcortical limbic areas (hippocampus, amygdala). MacLean argued the architecture of limbic areas is ill-suited for cognitive processes. The hippocampus, viewed by MacLean as the centerpiece of the limbic system and a central structure for emotional functions, is recognized as one of the key areas related to higher cognitive functions, such as declarative or explicit memory and spatial cognition. Efforts to define the system have failed. Connectivity with old cortex is a flawed criterion if old cortex is itself an unjustified notion. Connectivity with the hypo-thalamus once seemed plausible, since that was a way of distinguishing relevant and irrelevant cortical areas, as anatomical techniques improved, areas from the neocortex were also found to be connected with the hypothalamus, as were areas of the spinal cord, potentially extending the limbic system across the entire brain. Finally, and perhaps most important, there is no evidence that the limbic syst., however defined, functions as an integrated -system in the mediation of emotion. While specific areas of the limbic syst. contribute to some emotional functions, these areas do not do so because they belong to a limbic system that evolved to perform emotional functions. Relatively few limbic areas have been shown to contribute to emotional functions. As noted above, the hippocampus, the centerpiece of the limbic system theory of emotion, has been strongly implicated in cognitive functions but the evidence for a role in emotion is far less impressive.
The limbic syst. theory attempted to explain all emotions within a single anatomical concept. Contemporary researchers are more inclined to focus on the tasks designed to study the brain systems of specific emotions. This has been a more profitable empirical approach” [22].
Corballis MC, “The mirror- system provided a natural platform for the subsequent evolution of language. In nonhuman primates, the system provides for the understanding of biological action, and possibly for imitation, both prerequisites for language. I argue that language evolved from manual gestures, initially as a system of pantomime, with gestures gradually “conventionalizing” to assume more symbolic form. The evolution of the episodic memory and mental time travel, probably beginning with the genus Homo during the Pleistocene, created pressure for the system to “grammaticalize,” involving increased vocabulary necessary to refer to the episodes separated in time and place from the present, constructions such as tense to refer to time itself, and the generativity to construct future (and fictional) episodes. In parallel with grammaticalization, the language medium gradually incorporated facial and then vocal elements, culminating in autonomous speech (albeit accompanied still by manual gesture) in our own species, Homo sapiens” [23].
Charles C Horn, “Nausea -vomiting are important as biological systems for drug side effects, disease co-morbidities, and defenses against food -poisoning. Vomiting can serve the function of emptying a noxious chemical from the gut, and nausea appears to play a role in a conditioned response to avoid ingestion of offending substances. The sensory- pathways for nausea and vomiting, such as the gut and vestibular inputs, are generally defined but the problem of determining the brain’s final common pathway and central pattern generator for nausea and vomiting is largely unsolved. A neuro-physiological analysis of brain pathways provides an opportunity to more closely determine the neurobiology of nausea and vomiting and its prodromal signs (cold sweating, salivation).
Nausea - vomiting are commonly studied at pharmacological, behavioral, and psychological levels of analysis. These approaches are represented by a large literature of human clinical- research studies highlighting the efficacy of various anti-emetic agents. Extensive work has also been conducted to demonstrate that treatments for disease do not have negative effects, such as nausea and vomiting that might limit their clinical application. The current scarcity of research on the neurobiological basis of nausea and vomiting is striking considering its clinical importance. For example, at 2006 annual meeting of the Society for Neuroscience there were > 14,000 presentation abstracts but only 19 contained the words vomiting, emesis, or nausea.
This review paper presents nausea and vomiting in the evolutionary context of food intake (what is the adaptive nature of these systems?), discusses the relevance of this topic to today’s world, and addresses the current understanding of the brain circuitry that generates nausea/ vomiting.
Nausea and vomiting: a Defenses systems against food poisoning.
Animals possess special abilities for survival and many of these are used for the foraging and consumption of food. Food intake is a risky behavior leading to the exposure of internal organs to possible food-related ailments, including viral and bacterial infection, allergies, and food intolerance. An important survival problem is to determine which foods are safe and animals possess a hierarchy of sensory systems that help in food identification. Many spoiled foods can be identified using olfactory cues and taste is an effective intake deterrent when food is sour or bitter.
Smell and taste, the gatekeepers of the alimentary tract, are not always effective in detecting the quality of food, and nausea-vomiting, as additional mechanisms for dealing with an unhealthy meal, play a large role in subsequent levels of defense. Emesis, along with diarrhea, helps rid the gastrointestinal tract of dangerous ingested toxins. The vomiting response is present in many species, appearing in most vertebrates (including representative members of fish, amphibia, reptiles, birds, and mammals and at least one invertebrate, the gastropod pleurobanchaea. However, the broad assessment of the emetic response across species is hampered by the problem of distinguishing emesis from processes of regurgitation and rumination; emesis is functionally different and likely represents a more forceful ejection of the gastric contents.
Several commonly used laboratory animals appear to lack a vomiting response (rat, mouse, guinea pig, and rabbit). It is worth noting however that only a few strains of these species have been tested for emesis, using a limited set of stimuli, and it is unknown whether all members of these species lack the response. The possibility exists that rodents possess a degenerate “emetic” response rather than an absent one. There is an isolated report of “retching” in mice and rats have a gag reflex, which has similar features to a single retch, triggered by mechanical stimulation of the pharynx. There are structural differences in the rat and mouse esophagus and diaphragm that would make it difficult to generate the emetic response. Perhaps the vomiting response became an unneeded level of protection in rodents because they possess other efficient ways to deal with potential toxicosis, including a finely tuned ability to develop conditioned flavor aversions (CFA).
Nausea is an aversive experience that often accompanies the emesis, and is a distinct perception, different from pain or stress. Although a rare condition, vomiting can occur without nausea. Nausea is not simply the result of a low level of stimulation to the emetic system, which if only increased in intensity would result in vomiting. Counter-intuitively, nausea is more difficult to treat than emesis using anti-vomiting medications. The severity of drug-induced emesis (in ex. from cancer chemotherapy) can be controlled with anti-emetic medications, such as 5-HT3 and NK1 receptor antagonists; but nausea is still a persistent problem These facts suggest that nausea and vomiting are at least partially separate physiological processes. Arguably, nausea is the driving force behind development of CFA – thus providing the potent unconditioned stimulus to support a learned response to avoid consumption of foods which make us sick Unfortunately, nausea is difficult to study in laboratory animals but animal behavior (salivation, conditioned aversion), under conditions that make humans nauseated, suggests the presence of a unique aversive state.
Pregnancy-induced nausea and vomiting has an adaptive advantage. Importantly, the first trimester is a period of rapid fetal growth, and includes critically the development of the CNS, which is highly susceptible to the toxicosis. Pregnant women also appear to be picky eaters during this period and tend to avoid meat and fish products, which are more likely to contain pathogens that might harm the fetus. In humans, the presence of pregnancy-induced nausea and vomiting in the first trimester is correlated with a healthy pregnancy. It is only in rare cases that pregnancy-induced nausea and vomiting extends beyond this time interval, compromising the health of mother and fetus; a condition called hyperemesis gravidarum” [24].
Cloninger CR, The functional structure of self-aware consciousness in human beings is described based on evolution of human brain functions. Prior work on heritable temperament and character traits is extended to account for the quantum-like and holographic properties (i.e. parts elicit wholes) of self-aware consciousness. Cladistic analysis is used to identify the succession of ancestors leading to human beings. The functional capacities that emerge along this lineage of ancestors are described. The ecological- context in which each cladogenesis occurred is described to illustrate the shifting balance of evolution as a complex adaptive system. Comparative neuro-anatomy is reviewed to identify the brain structures and networks that emerged coincident with the emergent brain functions. Individual differences in human temperament traits were well developed in the common ancestor shared by reptiles and humans. Neocortical development in mammals proceeded in five major transitions: from the early reptiles to early mammals, early primates, simians, early Homo, and modern Homo sapiens. These transitions provide the foundation for human self-awareness related to sexuality, materiality, emotionality, intellectuality, and spirituality, respectively. The functional structure of human self-aware consciousness is concerned with regulation of five planes of being: sexuality, materiality, emotionality, intellectuality, and spirituality. Each plane elaborates neocortical functions organized around one of the 5 special senses. The interactions among these five planes gives rise to a 5 x 5 matrix of subplanes, which are functions that coarsely describe the focus of neocortical regulation. Each of these 25 neocortical functions regulates each of five basic motives or drives that can be measured as temperaments or basic emotions related to fear, anger, disgust, surprise, and happiness/sadness. The resulting 5 x 5 x 5 matrix of human characteristics provides a general and testable model of the functional structure of human consciousness that includes personality, physicality, emotionality, cognition, spirituality in a unified developmental framework [25].
Javier DeFelipe, “The tremendous expansion and the differentiation of the neo-cortex constitute 2 major events in the evolution of the mammalian -brain. The increase in size and complexity of our brains opened the way to a spectacular development of cognitive and mental skills. This expansion during evolution facilitated the addition of microcircuits with a similar basic structure, which increased the complexity of the human brain and contributed to its uniqueness. Fundamental differences even exist between distinct mammalian species. we shall discuss the issue of our humanity from a neurobiological and historical perspective.
Homo sum, humani nihil a me alienum puto (I am a human being, and therefore, nothing human is strange to me) Publius Terentius Afer (195/185–159 BC).
The nervous -system has evolved over millions of years, generating a wide variety of species-specific brains and behavioral capacities. The production and appreciation of art seems to be a uniquely human attribute, a recently acquired cognitive capacity in the genus Homo. Almost everything that the human being creates has a touch of art, although we do not need beauty or an esthetic perception to survive but rather, it just simply produces intellectual pleasure. The same occurs with other mental activities, like reading a book / listening to music. It seems obvious that only anatomically modern humans (Homo sapiens) can be behaviorally modern, capable of creating symbolic objects. Maybe this is when we discovered the world of ideas and created concept of the soul or spirit. From that moment, the relentless pursuit to define where such a trait is forged began, resulting in the so called “mind–body problem.” Of the numerous images available, we have chosen two here to illustrate in distinct ways the relationship between mental and physical worlds, both suggesting a separation between the two entities. Perhaps modern neuroscience has contributed most in this field by addressing the issue of mental processes from a biological standpoint. Nevertheless, it is striking how little influence this neuro-scientific knowledge has had on society due to the failure in conciliating the relationship between the brain and our humanity. It is commonly thought that increase in complexity as our brain has evolved is a product of the addition of microcircuits with a similar basic structure that incorporate only minor variations. Indeed, species-specific behaviors may arise from very small changes in neuronal- circuits. However, we will see that the human cerebral cortex has some distinctive circuits that are most likely related to our humanity. There are some erroneous popular beliefs regarding the relationship between brain size, evolution, and intellectual capabilities, and regarding the patterns of convolutions and the external morphology of the brain. Here, I shall deal with these topics with the aid of some historical notes” [26].
Jon H Kaas, “The large size and complex organization of the human brain makes it unique among primate brains. In particular, the neo-cortex constitutes about 80% of the brain, and this cortex is subdivided into a large number of functionally specialized regions, the cortical areas. Such a brain mediates accomplishments and abilities unmatched by any other species. How did such a brain evolve? Answers come from comparative studies of the brains of present-day mammals and other vertebrates in conjunction with information about brain sizes and shapes from the fossil record, research of brain development, and principles derived from studies of scaling and optimal design. Early mammals were small, with a small brains, an emphasis on olfaction, and little neo-cortex. Neo-cortex was transformed from the single layer of output pyramidal neurons of the dorsal cortex of earlier ancestors to the six layers of all present-day mammals. This small cap of neocortex was divided into 20–25 cortical areas, including primary and some secondary sensory areas that characterize neo-cortex in nearly all mammals today. Early placental mammals had a corpus callosum connecting the neo-cortex of the two hemispheres, a primary motor area, M1, and perhaps one or more premotor areas. One line of evolution, Euarchontoglires, led to present-day primates, tree shrews, flying lemurs, rodents and rabbits. Early primates evolved from small-brained, nocturnal, insect-eating mammals with an expanded region of temporal visual cortex. These early nocturnal- primates were adapted to the fine branch niche of the tropical rainforest by having an even more expanded visual system that mediated visually guided reaching and grasping of insects, small vertebrates, and fruits. Neo-cortex was greatly expanded, and included an array of cortical areas that characterize neo-cortex of all living primates. Specializations of the visual system included new visual areas that contributed to a dorsal stream of visuo-motor processing in a greatly enlarged region of posterior parietal cortex and an expanded motor system and the addition of a ventral premotor area. Higher visual areas in a large temporal lobe facilitated object recognition, and frontal cortex, included granular pre-frontal cortex. Auditory- cortex included the primary and secondary auditory areas that characterize the prosimian and anthropoid primates today. As anthropoids emerged as diurnal primates, the visual system specialized for detailed foveal- vision. Other adaptations included expansion of prefrontal cortex and insular cortex. The human and chimpanzee-bonobo lineages diverged some 6, 8 million years ago with brains that were about one-third the size of modern humans. Over the last 2 million years, the brains of our more recent ancestors increased greatly in size, especially in the prefrontal, posterior parietal, lateral temporal, and insular regions. Specialization of the 2 cerebral hemispheres for related, but different functions became pronounced, and language and other impressive cognitive abilities emerged” [27].
Harvey J Karten, “The organization of the non-mammalian forebrain had long puzzled neurobiologists. Unlike typical mammalian brains, the telencephalon is not organized in a laminated ‘cortical’ manner, with distinct cortical areas dedicated to individual sensory modalities or motor functions. The 2 major regions of the telencephalon, the basal ventricular ridge and the dorsal ventricular ridge, were loosely referred to as being akin to the mammalian basal ganglia. The telencephalon of non-mammalian vertebrates appears to consist of multiple ‘subcortical’ groups of cells. Analysis of the nuclear organization of the avian brain, its connections, molecular properties - physiology, and organization of its pattern of circuitry and function relative to that of mammals, collectively referred to as ‘evolutionary connectomics’, revealed that only a restricted portion of the BVR is homologous to the basal ganglia of mammals. The remaining dorsal regions of the DVR, wulst and arcopallium of the avian brain contain telencephalic inputs and outputs remarkably similar to those of the individual layers of the mammalian ‘neo-cortex’, hippocampus and amygdala, with instances of internuclear connections strikingly similar to those found between cortical layers and within radial ‘columns’ in the mammalian sensory and motor cortices. The molecular properties of these ‘nuclei’ in birds and reptiles are similar to those of the corresponding layers of the mammalian neo-cortex. The fundamental path-ways and cell groups of the auditory, visual and somatosensory systems of the thalamus and telencephalon are homologous at the cellular, circuit, network and gene levels, and are of great antiquity. A proposed altered migration of these homologous neurons and circuits during development is offered as a mechanism that may account for altered configuration of mammalian telencephalae” [28].
Robert K Naumann et al., “Deep inside the skull of every one of us there is something like a brain of a crocodile. Surrounding the R-complex is the limbic system or mammalian- brain, which evolved tens millions of years ago in ancestors who were mammal but not yet primates. It is a major source of our moods and emotions, of our concern and care for the young. Living in uneasy truce with the more primitive brains beneath, is cerebral cortex; civilization is a product of the cerebral cortex.”
“Carl Sagan’s amusing words of wisdom notwithstanding — is the H-bomb not also a product of the cerebral cortex? — is the reptilian brain really just a mammalian brain missing most of the parts? Some 320 million years ago, the evolution of a protective membrane surrounding the embryo, the amnion, enabled vertebrates to develop outside of water and thus to invade new terrestrial- niches. These amniotes were the ancestors of today’s mammals and sauropsids (reptiles, birds). Present-day reptiles are a diverse group of more than 10.000 species that comprise sphenodons (‘Tuatara’), lizards, snakes, turtles and the crocodilians. Although turtles were once thought to be the most ‘primitive’ among reptiles, current genomic data point toward two major groupings: the Squamata (lizards and snakes);a group comprising both the turtles and the Archosauria (dinosaurs, modern birds and crocodilians). Dinosaurs inhabited the Earth from the Triassic period (230 million years ago), at a time when the entire landmass formed a single Pangaea. They flourished from the beginning of the Jurassic to the mass extinction at the end of the Cretaceous (65 million years ago), and birds are their only survivors.
Vertebrate phylogeny, gross brain morphology, and homologous regions in the forebrain
Phylogenetic tree of vertebrates and timeline of major events in amniote-evolution. Shaded area in the phylogenetic tree indicates ‘reptiles’ as defined in the text. Red lines (lower panel) indicate the origin of amniotes (320 million years ago), the origin of dinosaurs (230 million years ago) and their extinction (65 million years ago). Schematic drawings showing brains (lateral view, anterior left) of vertebrate representatives: from top to bottom, a fish (knife-fish), an amphibian (tiger salamander), a reptile (monitor lizard), a bird (pigeon) and a mammal (hedgehog tenrec). Major subdivisions (examples in color) are present in all species but appear in different proportions. Transverse section of the right hemisphere of vertebrate representatives: from top to bottom, a fish (zebra fish), an amphibian (frog), a reptile (lizard), a bird (pigeon) and a mammal (rat). Colors represent conserved pallial subdivisions.
What we generally call reptiles is thus a group defined in part by exclusion: it gathers amniote species that are neither mammals nor birds, making the reptiles technically a paraphyletic grouping. Despite this technical point, the so-defined reptiles share many evolutionary, anatomical, developmental, physiological (for example, ectothermia), and functional features. It is thus reasonable to talk about a ‘reptilian brain’ — the subject of this Primer.
Structure and evolution of the reptilian brain
The diversity of reptiles and their evolutionary relationship to mammals make reptilian brains great models to explore questions related to the structural and functional evolution of vertebrate neural circuits. To this end, comparative research studies seek to identify homologies — structural or molecular similarities that are due to common ancestry — at a variety of levels, for example, brain regions, circuits or cell types. Homologies can be inferred from extant species by using a comparative approach within a phylogenetic framework. The Vertebrate brains have been classically compared in terms of morphology, connectivity, and neurochemistry; adult neuro-anatomy may not be sufficient to determine homologies without ambiguity.
Vertebrates share among themselves the highest morphological resemblance not as adults, but at their so-called ‘phylotypic -stage’ of embryonic development, a resemblance mirrored by similarity across transcriptomes. Identification of the conserved brain subdivisions, established by conserved signaling centers and uniquely defined by the combinatorial expression of transcription factors during development, demonstrates that all of the general brain regions found in mammals, including the cerebral cortex, have homologies in reptiles. The cerebral- cortex is part of the pallium, a developmental subdivision of telencephalon delineated by the expression of transcription factors such as Pax6, Emx1 and Tbr1, which is conserved in all vertebrates. Gene expression data show that the same fundamental subdivisions of the pallium — lateral, ventral, medial and dorsal, the latter giving rise to the neocortex in mammals — occur in developing vertebrates, despite the divergent morphologies of pallial structures in adults. The cerebral cortex is thus not a mammalian invention, but rather an ancient pallial structure that predates the split between sauropsids and theropsids, the mammals’ precursors.
Developmental and adult bauplan of the vertebrate brain.
Simplified developmental scheme of vertebrate brain regions. Combinatorial expression of transcription factors such as Tbr1, Dlx5, and Gbx2 defines brain regions during development and sets the stage for further differentiation in adults. Tbr1 and Dlx5 delineate further regions not shown here.
Lateral and medial pallium express different sets of molecular markers during development. Which regions of the adult brain correspond to lateral and medial pallium is an active topic of research and thus we combine both pallial subdivisions into a single region.
Comparative studies of brain structure and development have revealed a general Bauplan, an outline of the fundamental large-scale architecture of the vertebrate brain, reflecting its basic functional organization. The telencephalon integrates and stores multimodal information, and is also the higher center of action selection and motor control (basal ganglia). The hypothalamus is a conserved area controlling homeostasis and behaviors essential for survival, such as feeding and reproduction. In all vertebrates, behavioral states are controlled by common brainstem neuro-modulatory circuits, such as the serotoninergic system. Vertebrates harbor a diverse set of sense organs, and their brains share pathways for processing incoming sensory inputs. For example, in all vertebrates, visual information from the retina is relayed and processed to the pallium through the tectum and the thalamus, while olfactory input from the nose first reaches the olfactory bulb and then the pallium.
Although pallial structures exist in amphibians and fish, reptiles and mammals are the only vertebrates to have a cerebral- cortex with a clear, though simple, three-layered structure, similar to that of mammalian allocortex. The reptilian ventral pallium also gives rise to the dorsal ventricular ridge, a structure that dominates the bird pallium and contributes to the complex cognitive abilities of birds, but whose mammalian equivalent is still the subject of debate among comparative anatomists. The reptilian- cortex contains far fewer subdivisions than that of rodents, carnivores, or primates: it is subdivided into a medial cortex, often called hippocampus by anatomists; a lateral cortex, equivalent to the mammalian piriform cortex; and a dorsal cortex in between, which receives multimodal inputs (for example, visual inputs in turtles). There is evidence for motor and somatosensory areas in the reptilian cortex, pallial motor control may have evolved early in vertebrate evolution. Owing to this simplicity (Figure 3), the reptilian brain facilitates the study of primordial cortical function as a whole, and points to the origins of cortex as fulfilling general associative functions.
Visual circuits in monkey and turtle
Comparison of known visual cortical circuits between macaque and freshwater turtle, emphasizing the great simplicity of reptilian- cortex. Arrow indicates direction of information flow from sensory periphery. aDVR, anterior dorsal ventricular ridge (a: auditory, s: somatosensory, v: visual); dLGN, dorsal lateral geniculate nucleus; D1 and D2, dorsal cortex areas D1 and D2; DM, dorsomedial cortex; M (HC), medial cortex (‘hippocampus’); N. Rot., nucleus rotundus (‘pulvinar’); OT, optic tectum (‘superior colliculus’); RGC, retinal ganglion cells.
Besides sharing pallial modules, mammals and reptiles also share a complement of cortical cell types, suggesting that some structural elements of cortical -circuits arose early in amniote evolution. Like the mammalian- cortex, the reptilian cortex contains excitatory, glutamatergic neurons, inhibitory (GABAergic) interneurons. In both mammals and reptiles, these neurons have a common developmental origin: excitatory neurons are generated by multipotent cortical progenitors, whereas inhibitory neurons are born in subpallium before migrating to cortex.
Classical studies suggest that the reptilian main cortical cell layer (layer 2, L2) corresponds to the deep, output layers of mammalian neo-cortex, whereas its layer 1 (L1) is equivalent to mammalian layer I. According to this view, mammalian cortical evolution would have included the incorporation of new, intermediate cell layers acting as input stations and internal circuitry. Morphologically, L2 pyramidal neurons of the reptilian dorsal cortex are most similar to mammalian hippocampal excitatory- neurons. Indeed, reptilian pyramidal neurons have, depending on the area, little to no basal dendritic field, and several densely spine-studded apical dendrites, quite different from the single, long, apical dendrite of neocortical pyramidal neurons. Consistent with this correspondence between layers, reptilian sub-pallial cells transplanted into mammalian embryos generate GABA-ergic neurons that can populate only the deeper cortical layers.
Challenging this view recent molecular studies have found that turtle and lizard cortical neuro-blasts generate neurons that express upper layer molecular markers, in a developmental sequence similar to that observed in mammals. Although the molecular characterization of the neuronal types in the reptilian cortex is still in its infancy, it is possible that the reptilian cortex represents an ancestral blueprint for the more elaborate mammalian cortical circuits. Reptilian cortical- neurons, or subsets of them, might share molecular (and functional) features with both upper and lower layer mammalian cells.
Cortical circuitry is where most of the comparative work on the reptilian brain has been done. In the three-layered reptilian cortex, afferent inputs travel medially through superficial L1, where they fan out in a non-topographic manner. Their targets are GABA-ergic interneurons (with cell bodies in L1, L3) and glutamatergic pyramidal neurons, with cell bodies packed in L2, and dendrites in L1, L3. Interneurons project locally within cortex, providing feed forward and feedback inhibition to specific regions of the pyramidal cells’ dendrites. Several inter-neuron subtypes can be defined based on the expression of a subset of common genetic markers used in mammals. In turtle cortex, some interneuron types express calbindin, others express neuropeptide-Y, while parvalbumin (PV)-pos interneurons appear to be absent. Pyramidal cells make reciprocal connections with each other and with interneurons locally, with subcortical afferent structures, and with other cortical -areas.
The lateral cortex receives olfactory input from the olfactory bulb, and projects to the medial cortex (hippocampus). The dorsal cortex receives input from the thalamus; in many species this input is visual, originating in the thalamic lateral geniculate nucleus and also eventually reaches the hippocampus. Pyramidal cells of the hippocampus project back to dorsal and lateral cortices, forming an internal -cortical loop. Different mammalian species can exhibit vast elaborations in the number and connectivity of cortical subregions. By examining the homologous structures, shared circuit motifs can be recognized.
Shared circuit motifs between reptilian and mammalian cortex
Whereas processing steps are fewer in reptiles, they reach the same target as in mammalian cortex. Cortical architecture is more similar across regions in reptiles, suggesting a similar and possibly general computations are performed on different sensory inputs.
In the turtles, visual stimulation triggers propagating waves of neural activity that travel across the cortex. These waves are slower and simpler than those observed in mammalian neocortex. They are accompanied by relatively slow oscillations, which are most prominent in the 20 Hz frequency band. Whereas the so-called gamma oscillations in mammalian- cortex are typically around and above 40 Hz, recent results in mice indicate that the 20 Hz band dominates when PV-interneuron development is artificially arrested, consistent with the above observation that turtle cortex lacks PV inter-neurons. The computational role, if any, of such dynamics is unknown at present. Progress will require new experimental approaches that allow the simultaneous sampling of large neuronal populations. Specific and data-driven theories of computation in reptilian- cortex thus await further study. To the extent that modern reptilian cortex resembles that in the common ancestor of reptiles and mammals, understanding reptilian cortex function may reveal some of the fundamental associative computations that early cortical circuits evolved to carry out” [29].
Wynn T et al. write, “How did the human mind evolve? How and when did we come to think in the ways we do? This research has encompassed a range of biological and social sciences, from epigenetics /cognitive neuroscience to social - developmental psychology. Evolutionary scholars, including paleoanthropologists, have deployed the standard array of evolutionary methods. Ethological and experimental evidence has added significantly to our understanding of nonhuman brains and cognition, especially those of nonhuman primates. Studies of fossil brains through endocasts and sophisticated imaging techniques have revealed evolutionary changes in gross neural anatomy. Psychologists have also gotten into the game through application of reverse engineering to experimentally based descriptions of cognitive functions. For hominin evolution, there is another rich source of evidence of cognition, the archeological record. Using the methods of Paleolithic archeology and the theories and models of cognitive science, evolutionary cognitive archeology documents developments in the hominin mind that would otherwise be inaccessible” [30].
According Averof M et al., “2 hypotheses have been proposed for the origin of insect wings. One holds that wings evolved by modification of limb branches that were already present in multibranched ancestral appendages and probably functioned as gills. The second proposes that wings arose as novel outgrowths of the body wall, not directly related to any pre-existing limbs. If wings derive from dorsal- structures of multibranched appendages, we expect that some of their distinctive features will have been built on genetic functions that were already present in structural progenitors of insect wings, and in homologous structures of other arthropod limbs. We have isolated crustacean homologues of two genes that have wing-specific functions in insects, pdm (nubbin) and apterous. Their expression patterns support the hypothesis that insect wings evolved from gill-like appendages that were already present in the aquatic- ancestors of both crustaceans and insects” [31].
BJ Pearson et al. showed that, “All multi-cellular organisms have requirements for tumor suppression to regulate cellular proliferation during either embryonic development or adult life. Different organisms have vastly different requirements. Adult tumor suppression is probably not crucial to organisms possessing both short life spans and largely postmitotic soma. Animals with lifelong tissue turnover or those capable of regenerating body parts lost to injury must possess evolutionarily selected mechanisms to control rates of cell proliferation such that tissue homeostasis can be maintained or restored after injury. We hypothesize that these differences may help to explain why the lists of tumor suppressor genes in humans and Drosophila are largely nonoverlapping. We address this disparity by examining the tumor suppressor gene content of two outgroups to the vertebrates and flies/nematodes: the freshwater planarian and the single-celled choanoflagellate. Both organisms have recently had their genomes sequenced, giving us a first glimpse of which known tumor -suppressor genes have been maintained during evolution. In addition, we attempt to resolve which genes may have had ancestral tumor suppressor function and which may have acquired this function de novo” [32].
Hojung Nam et al., “Evolution results from molecular-level changes in an organism, thereby producing novel phenotypes and, eventually novel species. Changes in a single gene can lead to significant changes in biomolecular networks through the gain and loss of many molecular interactions. Thus, significant insights into microbial evolution have been gained through the analysis and comparison of reconstructed metabolic networks. However, challenges remain from reconstruction incompleteness and the inability to experiment with evolution on the timescale necessary for new species to arise. Despite these challenges, experimental laboratory evolution of microbes has provided some insights into the cellular objectives underlying evolution, under the constraints of nutrient availability and the use of mechanisms that protect from the extreme conditions.
While evolution involves the change of genetic sequence over time, it more importantly changes molecular interactions. Therefore, evolution often leads to modifications in molecular-network topology and ultimately in the systems-level functions of an organism (phenotype).
Metabolismis an ideal system to study network-level changes in evolution. It is the best characterized bio-molecular network. The underlying genes and proteins are well-characterized, and their interactions and biochemistry have been studied comprehensively. Because metabolism is critical to all cellular functions, many core reactions have ancient origins and are conserved across all kingdoms of life. While small differences exist in metabolic pathway topology within different strains of one species (in Pseudomonas syringae), examination of conserved reactions across species nonetheless facilitates clear comparisons for evolutionary insight.
Metabolismis an ideal system to study network-level changes in evolution. It is the best characterized bio-molecular network. The underlying genes and proteins are well-characterized, and their interactions and biochemistry have been studied comprehensively. Because metabolism is critical to all cellular functions, many core reactions have ancient origins and are conserved across all kingdoms of life. While small differences exist in metabolic pathway topology within different strains of one species (in Pseudomonas syringae), examination of conserved reactions across species nonetheless facilitates clear comparisons for evolutionary insight.
The Selective pressures resulting from cellular objectives help guide the expansion and pruning of metabolic pathways through evolution. The cellular objectives underlying the selective pressure can involve maximizing the nutritional potential of the environment and surviving environmental stress. Here we review recent insights into metabolic- pathway evolution, which have been gained from both computational and experimental approaches” [33] (Figures 5-9).
Figure 9: From Evolution, comparative biology and ontogeny of vertebrate heart regeneration. Celine J Vivien et al.
G Burnstock, “The anatomy of the autonomic nervous system in teleost fish, amphibia, reptiles, and birds is essentially similar to that seen in mammals. However, a sacral parasympathetic system is not present in teleost fish and urodele amphibians and is only rudimentary in anurans. In cyclostome fish there are no sympathetic chains or segmental sympathetic ganglia and in elasmobranchs there are series of paravertebral ganglia linked by a loose plexus of nerve bundles rather than a compact sympathetic chain, and grey rami are absent.
The vagal supply to visceral muscles such as the stomach and lung is primitively nonadrenergic and inhibitory. At some evolutionary stage higher than the Amphibia, the cholinergic excitatory function classically attributed to the vagus has been transferred from the sympathetic to the parasympathetic outflow. This may also be correlated with the switch from primitive sympathetic cholinergic excitatory supply of the viscera to predominantly adrenergic inhibitory control.
The primitive parasympathetic- innervation of the heart is cholinergic and inhibitory and this has been consistently retained throughout vertebrate evolution. Similarly adrenergic cardioaccelerator fibres appear early in evolution and are retained throughout. Primitively they run together with vagal fibres in the vago-sympathetic trunks and only at the reptile level reach the heart by separate nerve trunks, although the presence of a few adrenergic fibres of sympathetic origin still remain in the vagal trunks even in mammals. The presence of special catecholamine-containing cells appears to be a consistent feature of the vertebrate- heart.
Nerve trunks of the morphologically defined sympathetic nervous system in fish and amphibia consist of predominantly excitatory cholinergic fibres. There are various proportions of adrenergic and cholinergic fibres in sympathetic trunks in reptiles and birds, while in mammals, most, and sometimes all, of the fibres in sympathetic trunks are adrenergic. There has been an overall trend from cholinergic to adrenergic sympathetic control of visceral and vascular systems.
The development of preganglionic adrenergic fibres modulating the activity of nonadrenergic intramural ganglion cells in such organs as gut and bladder is not established until the mammals, although there is some rudimentary development of this kind in reptiles and birds.
The presence of intramural adrenergic nerve cells in various organs appears to be an evolutionary development that has taken place independently in many groups (e.g., lizard gut, toad lung, frog bladder). However it has not become established as a major feature of later evolution in mammals; no adrenergic cell- bodies have been found in the gut and few in the heart and bladder.
The influence of circulating catecholamines released from diffusely clistributed chromaffin cells appears to play a much more significant role in lower, than in higher vertebrates, in which direct nervous control has been developed to a more sophisticated degree. Chromaffin tissue is widespread in cyclostomes and is closely associated with segmentally arranged sympathetic ganglia in elasmobranchs and to a large extent in teleosts and amphibians” [34].
Taylor EW et al., “Heart rate in vertebrates is controlled by activity in autonomic nervous system. In spontaneously active or experimentally prepared animals, inhibitory parasympathetic control is predominant and is responsible for instantaneous changes in heart rate, such as occur at the first air breath following a period of apnoea in discontinuous breathers like inactive reptiles or species that surface to air breathe after a period of submersion. Parasympathetic-control, exerted via fast-conducting, myelinated efferent fibres in the vagus nerve, is also responsible for beat-to-beat changes in heart rate such as the high frequency components observed in spectral analysis of heart rate variability. These include respiratory modulation of the heartbeat that can generate cardio-respiratory synchrony in fish and respiratory sinus arrhythmia in mammals. Both may increase the effectiveness of respiratory gas exchange. Although the central interactions generating respiratory modulation of the heartbeat seem to be highly conserved through vertebrate phylogeny, they are different in kind and location, and in most species are as yet little understood. The heart in vertebrate embryos possesses both muscarinic cholinergic and β-adrenergic receptors very early in development. Adrenergic control by circulating catecholamines seems important throughout development. Innervation of the cardiac receptors is delayed and first evidence of a functional cholinergic tonus on the heart, exerted via the vagus nerve, is often seen shortly before or immediately after hatching or birth, suggesting that it may be coordinated with the onset of central respiratory rhythmicity and subsequent breathing” [35].
Celine J Vivien et al., “There are 64,000 living species of vertebrates on our planet and all of them have a heart. Comparative analyses devoted to understanding the regenerative potential of the myocardium have been performed in a dozen vertebrate species with the aim of developing regenerative- therapies for human heart disease. Based on this relatively small selection of animal models, important insights into the evolutionary conservation of regenerative mechanisms have been gained. We survey cardiac regeneration research in diverse species to provide an evolutionary context for the lack of regenerative capacity in the adult mammalian heart. Our analyses highlight the importance of cardiac adaptations that have occurred over hundreds of millions of years during the transition from aquatic to terrestrial life, as well as during the transition from the womb to an oxygen-rich environment at birth. We also discuss the evolution and ontogeny of cardiac morphological- physiological and metabolic adaptations in the context of heart regeneration. Our findings suggest that cardiac regenerative potential correlates with a low-metabolic state, the inability to regulate body temperature, low heart pressure, hypoxia, immature cardio-myocyte structure and an immature immune system. A more complete understanding of the evolutionary context and developmental mechanisms governing cardiac regenerative capacity would provide stronger scientific foundations for the translation of cardiac regeneration therapies into the clinic practice.
To date, comparative analyses devoted to understanding the regenerative potential of the heart have been performed in a dozen or so vertebrate species. In teleost fish, heart regeneration occurs during the larval and adult life, although regenerative mechanisms differ depending on developmental stage and type of injury. For reasons that are currently unclear, some adult teleosts, such as medaka, cannot undergo cardiac regeneration. It is unclear whether cardiac regenerative ability was lost in medaka during its evolutionary divergence from zebra fish or whether zebra fish gained the genetic networks required for regeneration. In amphibians, heart regeneration occurs during the aquatic life but, to date, cardiac regeneration has not been documented in terrestrial amphibians. Cardiac regeneration in mammals appears to be restricted during a defined early-developmental period during embryonic and early-neonatal life. The genetic and environmental causes of these evolutionary transitions over hundreds of millions of years of evolution remain poorly defined.
In the most vertebrate species, cardiac regenerative potential seems to correlate with a low-metabolic state, low heart pressure, immature- cardiomyocyte structure, hypoxia, an immature immune system and the inability to regulate body temperature. The relationship between these physiological parameters and the ability to regenerate is not well-understood. From an evolutionary perspective, it will be important to assess heart -regeneration in a more diverse array of species including birds, reptiles and marsupials. Such comparative analyses would enable the identification of core evolutionarily conserved mechanisms for vertebrate heart regeneration and provide a more nuanced understanding of the role of genes and the environment in the regulation of this complex process.
Cardiac regenerative ability is a highly variable trait that exists in diverse species and at different stages of development in multiple vertebrates across the animal kingdom. Understanding the evolutionary context and mechanisms that govern cardiac regenerative capacity in mammals during early stages of development would provide stronger scientific foundations for the translation of cardiac regeneration therapies into the clinic” [36].
Keyue Ding et al., “Susceptibility to common diseases such as coronary heart disease (CHD) may in part reflect historical or evolutionary legacies, and interest in studying evolutionary biology to gain novel insights into human health and disease is increasing.
The evolutionary history of the human species may provide valuable insights into the origin of common diseases beyond what is possible by investigating only the most immediate or “proximal” causes of disease. The potential role of evolutionary biology in explaining disease causation was highlighted by Williams and Nesse and is often referred to as Darwinian- medicine. Although the relevance of an evolutionary perspective may vary depending on the disease under study, a strong argument could be made for studying the evolutionary genetics of CHD, a leading cause of human morbidity and death.
Within the last decade, several important advances have made it possible to study “modern” diseases from an evolutionary perspective. The Human Genome Project5 provided a reference human genome, and the subsequent International HapMap Project described genetic variations (mostly single nucleotide polymorphisms [SNPs]) among individuals and the patterns of variation across the genome. Both projects provide the raw material to study natural selection in the human genome, as reviewed in recent reports. Sequencing of multiple other genomes, including those of primates, provides a framework for generating important insights into the origin and expression of human diseases.
Such an avenue of investigation may help answer why, compared with their closest relative, the chimpanzee, humans are susceptible to CHD, why CHD has assumed epidemic proportions, and why substantial differences in susceptibility to CHD are present between ethnic groups. Genetic factors underlying both “complex” and mendelian diseases may be affected by natural selection; conversely, regions of the human genome under natural selection are likely to harbor functional loci (gene coding and regulatory regions) that may influence disease susceptibility. Inferences about natural selection may therefore help identify disease susceptibility loci in humans and facilitate disease mapping studies.
In this review, we discuss the current hypotheses and knowledge about the evolutionary genetics of several risk factors for CHD, including hypertension, dyslipidemia, and metabolic syndrome. Several candidate genes in pathways of blood pressure, glucose and lipid metabolism, blood coagulation, and inflammation that may be under natural selection are enumerated. An evolutionary perspective might explain why contemporary humans are at high risk for CHD and also helps to better understand the variation in disease susceptibility” [37].
Mei Chen et al., “Mammalian platelets are small, anuclear circulating cells that form tightly adherent, shear-resistant thrombi to prevent blood loss after vessel injury. Platelet thrombi that form in coronary and carotid arteries also underlie common vascular diseases such as myocardial infarction IM and stroke and are the target of drugs used to treat these pathology. Birds have high-pressure cardiovascular systems like mammals but generate nucleated thrombocytes rather than platelets. Here, we show that avian thrombocytes respond to many of the same activating stimuli as mammalian platelets but are unable to form shear-resistant aggregates ex vivo. Avian thrombocytes are larger than the mammalian platelets, spread less efficiently on collagen, and express much lower levels of the 2b3 integrin required for aggregate formation, features predicted to make thrombocyte aggregates less resistant than platelets are to the high fluid shear forces of the arterial vasculature. In vivo carotid vessel injury stimulates the formation of occlusive platelet thrombi in mice but not in the size- and flow-matched carotid artery of the Australian budgerigar. These research studies indicate that unique physical and molecular features of mammalian platelets enable them to form shear-resistant arterial thrombi, an essential element in the pathogenesis of human cardiovascular- diseases.
The biology of platelets has been studied in great detail in the context of human disease and experimentally by the use of genetically modified mice, but little is known regarding the extent to which the hemostatic function of platelets is conserved with that of analogous cell types in other vertebrate species. We focused on avian thrombocytes and arterial thrombosis because platelets are thought to be causal for pathologic thrombus formation in human arteries and birds have blood pressures and cardiovascular physiologies that closely resemble those of mammals. Our research studies reveal that the basic molecular machinery of cellular hemostasis (eg, activation by thrombin and collagen) was established before the evolution of mammals and platelets but that mammalian platelets exhibit molecular and cellular differences from thrombocytes that have significant effects on the ability to form arterial thrombi. The 2 most important of these appear to be enhanced adhesion and spreading under shear, perhaps associated with loss of the cell nucleus, and the ability to form platelet aggregates that are highly stable and shear resistant. These platelet responses are likely to reflect high expression and/or increased affinity of the 2b3 integrin and the function of receptors such as P2RY12 that maintain the activation state of that integrin. These findings suggest a potential connection between the development of platelets in mammals and the pathogenesis of common human cardiovascular diseases.
Anuclear platelets are a distinguishing characteristic of all mammals, but why platelets evolved has not been clear. It has been proposed that platelets arose in association with the placentation and live birth, but platelets are present in egg-laying monotremes and therefore appeared early in mammalian evolution and many millions of years before live birth. The fossil record suggests that during the first 140 million years of the mammalian evolution, when dinosaurs (the ancestors of modern birds) were the dominant land animals, mammals were small, burrowing animals. Our research studies identify important functional differences between mammalian platelets and avian thrombocytes, eg, their small size, ability to change shape unimpeded by a cell nucleus, and molecular refinements such as a greater density of 2b3 integrins, that are consistent with more efficient hemostatic responses by platelets compared with thrombocytes. Although this prediction cannot be tested in all contexts, the finding that equivalent degrees of arterial vessel wall injury in vessels of similar size and equal hemo-dynamic forces result in the occlusion in mammals but not in birds is consistent with the hypothesis that platelets mediate more efficient thrombotic responses than nucleated thrombocytes. Thus, although the reason for platelet evolution in mammals can never be known with certainty, it is tempting to speculate that platelets may have allowed early mammals to better survive trauma and thereby provided a survival advantage.
The finding that chicken thrombocytes adhere to collagen under flow but are unable to form 3-dimensional aggregates like platelets do suggested that the shear-resistant platelet thrombi that form in human coronary arteries might not reflect a universal hemostatic response to arterial vessel wall injury. Indeed, FeCl3 injury of the carotid vessel wall of the mouse resulted in the formation of an occlusive platelet thrombus, even when the injury is limited to the area at the vessel wall. Identical injury of the size and flow-matched Australian budgerigar resulted in formation of a small mural thrombus that failed to propagate unless the FeCl3 injury extended to cells throughout the thrombus and vessel- lumen.
Finally, it is important to note that our studies address cellmediated arterial thrombosis and do not compare the relative contribution of platelets and thrombocytes to all aspects of hemostasis in vivo. The small mural thrombi formed by avian- thrombocytes may be sufficient to prevent excess blood loss after traumatic injury; alternatively, birds may use other mechanisms to support hemostasis.
Regardless, these studies suggest that the development of shear-resistant, vaso-occlusive arterial thrombi, a key step in the pathogenesis of myocardial infarction, is not a universal hemostatic response but instead depends on molecular and cellular features that evolved solely in mammalian platelets. Two of these features, high levels of the 2b3 integrin and expression of the P2RY12 receptor, are indeed the targets of primary antiplatelet drugs used to treat acute myocardial infarction. Thus, cardiovascular pathology in modern man may be linked to evolutionary changes that augmented hemostatic responses in early mammals” [38].
Richard L et al., “Rodents, non-human primates, dogs, cats, and farm animals have long been the preferred animal models in biomedical research. Their behavioral repertoire and physiological responses to stimuli are more similar to human systems than are lower organisms. However, as amphibians, fish and reptiles become well understood in the field and laboratory, their advantages as alternative animal models are being revealed species of amphibian, 6,000 species of reptiles, and more than 20,000 species of fish.
Such diversity allows for a wide range of research on specific organs, tissues, and systems that are similar in function, but often less complex than in mammals. Due to differential rates of ova maturation and numbers of gametes produced, elasmobranchs (sharks, skates, rays) are used to study fetal nutrition and toxicology as well as gametogenesis. The monkfish is an important insulin resource for researchers since it contains 20 to 40 times more insulin-producing tissue than the laboratory rat.
Reptiles and amphibians contribute to studies in genetics, embryology, developmental biology, ethology, endocrinology, and immunology. Salamanders and newts are models for limb regeneration. Fetal heart cells of the axolotl (Mexican salamander) will divide and beat in vitro. Frogs have played a major role in the development of communication theory. The common anole lizard is used in behavioral studies and can be induced to produce the symptoms of Parkinson=s disease. Typical reptile and amphibian studies include aggression, stress physiology, reproductive cycles and dysfunction, and the effects of neurotoxins.
In contrast to mammals and birds which are endothermic (their body temperature is regulated and maintained internally), reptiles, amphibians, and fish are ectothermic with body temperature primarily regulated by ambient temperatures. Since metabolism is driven by body temperature, the rate of normal body functions in these species may be artificially increased or decreased. Thus endocrine, immunological, inflammation, cell physiology, and many other pathways can be easily manipulated” [39].
Discussion
Related the results of reporter scientific literature analyzed in a unic way we propose to the scientific world to introduce a new discipline named ENDOGENOUS ARCHEOLOGY.
To collect and rationalize all the knowledge related to this topics to create a unic database and Source of information for future investigations.
Residual organs, genes, metabolic pathways, immune response, ancestral systems can be observed in actual human being as an archeological ruins to testify an evolutive process.
Emotional control, fear, aggressivity, sexual and surviving instinct, some immune process, onco suppressor, cardio- vascular functions and many other Functions are strictly related with a common evolution and with real objectively evidence.
Conclusion
Related to the result f this review- research work is possible to submit to the researcher community a new systematic discipline to collect, rationalize and join many previous and actual distinct theory in a new discipline.
A new discipline that can make possible to open at new research modalities Archeological evidence inside us to be managed as archerological finding Evidence like emotional management by humans are strictly related to the neuro- receptorial profile Can be used as traceant to investigate ancestral circuits.
A starting and in progress researchgate project (opened in November 2018) can be the right instrument to rationalize all scientific evidence produced since this time for future research and next practice applications.
A deep understand in our past can be really useful in scientific new application in many different field like Behavioral science, psychological but also in biology and medicine, pharmacology, immunology, cardio vascular, neurology and other relevant field and also in order to introduce new diagnostic or therapeutics strategies.
The use of a Filogenetic and Archeo-biological endogenous approach make possible to achieve better scientific results in many discipline useful to be translated in practice applications (new pharmacologic strategies for relevant disease).
Clarifications
This work is produced without any diagnostic or therapeutic intent, only to suggest new research hypotesys and methods.
References
- Luisetto M, Khan FA, Cabianca L, Mokbul MI, Rafa AY, et al. Amygdala pharmacology and crime behavior, dysfunctions to be considered as a disease. Int Arch BioMed Clin Res. 2016; 2: 1-4. Ref.: https://goo.gl/iyg5Jh
- Luisetto M, Mashori GR, Nili-Ahmadabadi B, Khan FA, Khan KR. Mindset kinetics and some depression status: A new quantitative model under biochemical - toxicology approach? Insights Depress Anxiety. 2018; 2: 029-039. Ref.: https://goo.gl/TnCkst
- Luisetto M. Brain and Transmission Signal Modulation. theranostic brain disorder 2017;
- Luisetto M. Jurisdictional Consequences in some brain condition. Neurochem Neuropharm 2017; 3: 2(Suppl). Ref.: https://goo.gl/yu1NXa
- Luisetto M, Nili-Ahmadabadi B, Mashori GR, Rafa AY, Sahu RK, et al. Brain and Immune System: KURU, a Strange Kind of Disease. An Endogenous Toxicological Process Like? EC Neurology. 2018; 10: 7. 613-625. Ref.: https://goo.gl/49b9AE
- Carey HV, Andrews MT, Martin SL. Mammalian hibernation: cellular and molecular responses to depressed metabolism and low temperature. Physiol Rev. 2003; 83: 1153-1181. Ref.: https://goo.gl/cYm1gV
- Davidson I. The archeology of cognitive evolution. Wiley Interdiscip Rev Cogn Sci. 2010; 1: 214-229. Ref.: https://goo.gl/suxux3
- Neubauer S, Hublin JJ, Gunz P. The evolution of modern human brain shape. Sci Adv. 2018; 4: eaao5961. Ref.: https://goo.gl/w5NrYR
- Bonett RM. Analyzing endocrine system conservation and evolution. Gen Comp Endocrinol. 2016; 234: 3-9. Ref.: https://goo.gl/ufMEuy
- Nunn CL, Samson DR, Krystal AD. Shining evolutionary light on human sleep and sleep disorders. Evol Med Public Health. 2016; 2016: 227–243. Ref.: https://goo.gl/dFTeZY
- Müller M. Energy metabolism of ancestral eukaryotes: a hypothesis based on the biochemistry of amitochondriate parasitic protists. Biosystems. 1992; 28: 33-40. Ref.: https://goo.gl/AN9U4B
- Fondi M, Brilli M, Emiliani G, Paffetti D, Fani R. The primordial metabolism: an ancestral interconnection between leucine, arginine, and lysine biosynthesis. BMC Evol Biol. 2007; 7(Suppl 2): S3. Ref.: https://goo.gl/kv8jTM
- Farhud DD, Yeganeh MZ. Brief History of Human Blood Groups. Iran J Public Health. 2013; 42: 1–6. Ref.: https://goo.gl/PNrRzE
- de Boer B. Evolution of speech and evolution of language. Psychon Bull Rev. 2017; 24:158-162. Ref.: https://goo.gl/hA6wwU
- Heyes C. New thinking: the evolution of human cognition. Philos Trans R Soc Lond B Biol Sci. 2012; 367: 2091–2096. Ref.: https://goo.gl/WGxueV
- Thrall PH, Bever JD, Burdon JJ. Evolutionary change in agriculture: the past, present and future. Evol Appl. 2010; 3: 405–408. Ref.: https://goo.gl/SsvTSS
- Luca F, Perry GH, Di Rienzo A. Evolutionary Adaptations to Dietary Changes. Annu Rev Nutr. 2010; 30: 291–314. Ref.: https://goo.gl/vY8vM9
- Tattersall I. Human evolution and cognition. Theory Biosci. 2010; 129: 193-201. Ref.: https://goo.gl/m7TBEZ
- Bar-Rogovsky H, Hugenmatter A, Tawfik DS. The evolutionary origins of detoxifying enzymes: the mammalian serum paraoxonases (PONs) relate to bacterial homoserine lactonases. J Biol Chem. 2013; 288: 23914-23927. Ref.: https://goo.gl/eMTptz
- Gehring WJ. The evolution of vision. Wiley Interdiscip Rev Dev Biol. 2014; 3: 1-40. Ref.: https://goo.gl/fuVznB
- Ko YA, Susztak K. Epigenomics: The science of no-longer-“junk” DNA. Why study it in chronic kidney disease? Semin Nephrol. 2013; 33: 354-362. Ref.: https://goo.gl/25n2gc
- LeDoux JE. Evolution of human emotion: A View through Fear. Prog Brain Res. 2012; 195: 431-442. Ref.: https://goo.gl/wJ5KLW
- Corballis MC. Mirror neurons and the evolution of language. Brain Lang. 2010; 112: 25-35. Ref.: https://goo.gl/zLaAFj
- Horn CC. Why is the neurobiology of nausea and vomiting so important? Appetite. 2008; 50: 430–434. Ref.: https://goo.gl/uvyfBa
- Cloninger CR. Evolution of human brain functions: the functional structure of human consciousness. Aust N Z J Psychiatry. 2009; 43: 994-1006. Ref.: https://goo.gl/CUVQCg
- DeFelipe J. The Evolution of the Brain, the Human Nature of Cortical Circuits, and Intellectual Creativity. Front Neuroanat. 2011; 5: 29. Ref.: https://goo.gl/JKksxz
- Kaas JH. The Evolution of Brains from Early Mammals to Humans. Wiley Interdiscip Rev Cogn Sci. 2013; 4: 33–45. Ref.: https://goo.gl/MuwQW5
- Karten hj. Vertebrate brains and evolutionary connectomics: on the origins of the mammalian ‘neocortex.’ Philos Trans R Soc Lond B Biol Sci. 2015; 370: 20150060. Ref.: https://goo.gl/8xN4Fm
- Naumann RK, Ondracek JM, Reiter S, Shein-Idelson M, Tosches MA, et al. The reptilian brain. Curr Biol. 2015; 25: R317–R321. Ref.: https://goo.gl/97ioZ9
- Wynn T, Coolidge FL. Archeological insights into hominin cognitive evolution. Evol Anthropol. 2016; 25: 200-213. Ref.: https://goo.gl/Ug9oZw
- Averof M, Cohen SM. Evolutionary origin of insect wings from ancestral gills. Nature. 1997; 385: 627-630. Ref.: https://goo.gl/HUoHSy
- Pearson BJ, Sánchez Alvarado A. Regeneration, Stem Cells, and the Evolution Tumor Suppression. Cold Spring Harb Symp Quant Biol. 2008; 73: 565-572. Ref.: https://goo.gl/UYXHjP
- Nam N, Conrad T, Lewis NE. Metabolic pathway evolution and cellular objectives. Curr Opin Biotechnol. 2011; 22: 595–600. Ref.: https://goo.gl/Eqr3CM
- G. BURNSTOCK. Evolution of the autonomic innervation of visceral and cardiovascular systems in vertebrates. Pharmacol Rev. 1969; 21: 247-324. Ref.: https://goo.gl/8p2Kf9
- Taylor EW, Leite CA, Sartori MR, Wang T, Abe AS, et al. The phylogeny and ontogeny of autonomic control of the heart and cardiorespiratory interactions in vertebrates. J Exp Biol. 2014; 217: 690-703. Ref.: https://goo.gl/SUsgYv
- Vivien CJ, Hudson JE, Porrello ER. Evolution, comparative biology and ontogeny of vertebrate heart regeneration. npj Regenerative Medicine. 2016; 16012. Ref.: https://goo.gl/9NbDZc
- Ding K, Kullo IJ. Evolutionary Genetics of Coronary Heart Disease. Circulation. 2009; 119: 459-467. Ref.: https://goo.gl/gBFHZx
- Schmaier AA, Stalker TJ, Runge JJ, Lee D, Nagaswami C, et al. Occlusive thrombi arise in mammals but not birds in response to arterial injury: evolutionary insight into human cardiovascular disease. Blood. 2011; 118: 3661-3669. Ref.: https://goo.gl/tyWwzx
- Crawford RL, Jensen D, Allen T. Information Resources for Reptiles, Amphibians, Fish, & Used in Biomedical Research. 2001; Information Resources on Amphibians, Fish & Reptiles. AWIC Resource Series No. 10. Ref.: https://goo.gl/VA5fGb