Review Article
Vigour of CRISPR/Cas9 Gene Editing in Alzheimer’s Disease
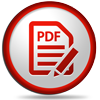
Jes Paul*
Department of Molecular and Cellular Physiology, Albany Medical College, Albany, NY, USA
*Address for Correspondence: Jes Paul, Department of Molecular and Cellular Physiology, Albany Medical College, Albany, NY, USA, Tel: 4049802103; Email: [email protected]; [email protected]
Dates: Submitted: 14 September 2018; Approved: 04 October 2018; Published: 05 October 2018
How to cite this article: Paul J. Vigour of CRISPR/Cas9 Gene Editing in Alzheimer’s Disease. J Neurosci Neurol Disord. 2018; 2: 047-051. DOI: 10.29328/journal.jnnd.1001014
Copyright License: © 2018 Paul J. This is an open access article distributed under the Creative Commons Attribution License, which permits unrestricted use, distribution, and reproduction in any medium, provided the original work is properly cited.
Keywords: Alzheimer’s disease; CRISPR/Cas9; Gene editing; Treatment; Huntington’s disease; iPSC neurons
Abstract
Ailment repairing regiments has turn out to be arduous, despite a plenty of understanding and knowledge acquired in the past relating to the molecular underpinnings of Alzheimer’s disease (AD. Umpteen clinical experiments targeting the fabrication and accumulation have been turned fruitless to fit potency standards. The tests aiming beta-amyloid hypothesis also turned futile making it exigent for further handling tactics. The new emanation of a comparably candid, economical, and punctilious system known as gene editing have showed light in path of cure for AD by CRISPR/Cas9 gene editing. Being a straight approach this procedure has already shown assurance in other neurological disorders too such as Huntington’s disease. This review standpoint the immanent service of CRISPR/Cas9 as a remedial option for AD by aiming on specific genes inclusive of those that induce early-onset AD, as well as those that are substantial risk components for late-onset AD such as the apolipoprotein E4 (APOE4) gene.
Preamble on Alzheimer’s Disease (AD)
Advancing and lethal neurodegenerative malady that chiefly affects older adults and is also the habitual root of dementia [1]. In clinical situation, AD is exhibited with guilefuly progressive memory loss to which other circles of cognition are eventually flawed. Language hindrances and missing of executive skills are the symptoms that typifies the term dementia. In kernel, AD impedes with remembrance, thoughts, and behavior rigorous enough to affect a person’s work, hobbies, and social life. AD is remorselessly progressive and deadly within 5 to 10 years [2]. An extracellular imbecile plaque constitutes of beta-amyloid and an intracellular lesion of shortened and hyper-phosphorylated TAU leading to neurofibrillary tangles (NFTs) is the foremost archetypal neuro pathological imprints of AD. The missing of synapses is straightly linked to the degree of dementia [3-5]. Early aggregation of beta-amyloid on PET scans allude to, feasibly, a vast salutary window to step in the clew instigating species, proceeding to all downstream events winding up in synapse loss, neurodegeneration and ultimately dementia is the beta-amyloid in the form of toxic oligomers [6-9].
The stimulus for the budding and analyzing of the first known disease-correcting therapeutics that conjointly had a deed of either prohibiting beta-amyloid from shaping or led to amplified clearance out of the brain is provided by the approach of the beta-amyloid hypothesis [10]. Regardless of the numerous number of clinical studies on AD, many are in vain. There are many ostensible reasons for these clinical trial failures. Due to the numerous failures recorded for the current disease-tweaking approaches, now may be time to solemnly convergence on other inherent treatment criterion including gene editing systems. Zinc finger nucleases (ZFNs), Transcription activator-like Effectors Nucleases (TALENs) and the CRISPR-associated nuclease 9 (CRISPR/Cas9) are the three gene editing tools procurable for AD. However, this review will focus on the benefit and avail of the CRISPR/Cas9 because it is quicker, affordable, more veracious and effectual than other present genome editing methods.
CRISPR/Cas9, a revolutionary genome editing tool
From the bacterial Type II CRISPR adaptive immune system, the RNA-guided agglomerated customarily interspaced short palindrome repeats/CRISPR associated nuclease 9 (CRISPR/Cas9) systems is extracted [11,12]. Targeting and slicing specific DNA sequences using only a nuclease and make RNAs to aim specific DNA sequences is the task of The CRISPR/Cas9 system. In bacteria, its chief role is endo-nucleolytic demolition of attacking plasmid or phage DNA [13]. A very brief preserved sequence is spotted by Cas9 ,nearby to the guide spacer sequence known as “Protospacer Adjacent Motif” (PAM).The attribute of the sequences is that they all share selfsame sequences at one end and uniform homology to viral genes. These sequences are necessary for target realization upon reaching the DNA target site, a double strand break followed by gene knockout effects of template-dependent gene replacement befall [14]. A small pre-designed RNA sequence positioned within a longer RNA scaffold known as guide RNA is the guide to the system.The predesigned sequence then counselor Cas9 to the right part of the genome, after that the scaffold part binds to DNA. [15]. At this point, the cleaved DNA is acknowledged by DNA repair mechanisms and is remodeled by cellular DNA repair mechanisms. Ease of use, low price, and its dexterity to beget gene knock-outs, knock-ins, or smaller mutations are the merits of the CRISPR/Cas9 system over others. Not only that they are able to sharply and skillfully target, edit, modify, monitor, and stamp genomic loci of a wide array of cells and organisms. The virtual utilization of CRISPR are long way and can empower large-scale screening for drug victims and other phenotypes and will promote the formation of engineered animal models that will be hoof the understanding of human diseases. The utmost aim of this system would be to rectify alterations at punctilious locations in the human genome in order to treat and handle genetic causes of disease inclusive of certain neurodegenerative disorders.
CRISPR/Cas9 in diverse neurological disorders
One of the neurological disorders where CRISPR/Cas9 technique has shown promise is Huntington disease (HD).The elaboration of CAG repeats in exon 1 of the HTT gene, which ciphers for mutant hunting in (mHTT), a large protein consisting of large polyglutamine repeats in the N-terminal domain of mHTT that most likely features a toxic-gain of function ensuing in HD. To treat HD one dormant tactics is by using CRISPR/Cas9 to selectively quell the expression of mHTT [16,17]. Despite some off target effects while using Cas9, recently a study investigated a novel variant of the gene-editing system that emerge to be prudent and clear-cut by using a nickase version of Cas9 [18].
This edition of Cas9 is favorable as it cuts just one DNA strand instead of two, which magnify the fidelity with which Cas9 can edit sequences of DNA. In essence, these discoveries cherish the convenience of using CRISPR/Cas9 to set right mutant protein expression in specific brain regions and opens up the potentialities of a novel treatment plans not only for HD but other neurodegenerative ailments that originates from mutant genes, including AD.
CRISPR/Cas9 in treating alzheimer’s disease
Recent studies of CRISPR/Cas9 on AD: The utility of CRISPR/Cas9 would not seem a doable therapeutic approach as the most of AD cases are spasmodic with the spark unknown. Mutations in the APP protein or genes products entangled in refining APP to form beta-amyloid is one the known mutations in AD. But only very few percentage of these cases (<1%) are taking place. Although these alterations make up a small percentage of known AD cases, they all allude to elevated synthesis of the beta-amyloid peptide [10]. Early-onset AD can betide due to other known mutations like presenilin 1 (PSEN1) and presenilin 2 (PSEN2) [19,20], which also tend to heighten the manufacture of beta-amyloid mayhap by switching the spitting site in APP [21]. All these occur before age 60 and is classified as early-onset AD. To rectify these autosomal-dominant deviations CRISPR/cas9 can be used. This is affirmed by fresh studies that have scrutinized the capacity of correcting similar kinds of changes using this gene editing system. Previous studies have also employed CRISPR/Cas9 to set right genetic AD mutations in the PSEN gene in patient-derived iPSCs [22,23]. Also a 60% reduction in exudation of beta-amyloid by CRISPR/Cas9 treatment is established by a novel study on Swedish APP mutation in patient-derived fibroblasts [24].
In addition to that, researches are being done in Sporadic AD models to edit endogenous APP at the distant C-terminus to abate β-cleavage and beta-amyloid origination [25] using CRISPR/Cas9 editing. The motive for this approach was tripping off the C-terminal region of APP forbids a sequentialinterplay with BACE-1 within endosomes, which is the first crucial cleavage event in giving rise to beta-amyloid [26]. Their modern findings in cell lines, cultured neurons, human iPSC-neurons and mouse brains all manifested the approach by confining the somatic association of APP with BACE-1 and debilitate beta-amyloid creation [25].
Moreover, the late-onset AD is shielding the apolipoprotein E4 (APOE4) allele [27], which is a major jeopardy as Human apoE is polymorphic with three significant isoforms, apoE2, apoE3, and apoE4, all of which vary by single amino acid replacement comprising cysteine-arginine replacements at positions 112 and 158 [28]. Among the three, the E2 allele is the infrequent form of APOE. Bearing even one copy of E2 appears to abridge the stake of evolving Alzheimer’s by up to 40%. The APOE3 is the most frequent allele and it doesn’t seem to affect risk. Roughly10–15% of the people, APOE4 is present, elevating the stake for AD and menacing the age of onset [29]. Having one copy of E4 (E3/E4) can amplify the risk 2 to 3 times, while two copies of E4 (E4/E4) can magnify the risk by 10–15 times [27]. At least one APOE4 allele is seen in 65–80% of all AD patients and it is striking [29,30]. Lastly, in spite of many of the inimical effects of APOE4 it seem to be linked with beta-amyloid [31]. A recent study suggest that apoE4 may boost pathology such as tau phosphorylation in human iPSC-derived neurons, self-reliant of beta-amyloid [32]. The authors also revealed that transforming apoE4 to apoE3 by gene editing (using zinc-finger nuclease-mediated gene editing not CRISPR/Cas9) thwarted the pathology linked with apoE4 in their model system [32]. Therefore, one potential utility of the CRISPR/Cas9 system could be to reform APOE4 to APOE2 or E3. In this respect constitutional and operational variations from apoE4 to apoE3 or apoE2 interceded through CRISPR/Cas9 may be a feasible approach to handle AD patients holding APOE4. Intriguingly, although amino acid residues 112 and 158 (cysteine to arginine substitutions) ascertain the diverse isoforms of APOE, proofshints a major structural trait of apoE4 that discern it from apoE2 and apoE3 is a domain synergy intervened by a salt bridge between Arg-61 and Glu-255 [33]. For this reason, the usage of CRISPR/Cas9 to modify any one of these two amino acids may also be as cogent in nullifying the risk allied with sheltering the APOE4 allele.
Conclusion
CRISPR/Cas9 can aim well-nigh any gene in a sequence-dependent manner, and its pointing proficiency is superior to other gene targeting techniques. Thus, this approach could be devoted to any quantity of autosomal-dominant mutations that steer to early-onset AD, or for those genetic risk factors that intensify the dementia risk kindred with late-onset AD, in particular the APOE4 allele. Nevertheless, dares including off-target consequences and aiming CRISPR/Cas9 to particular cell types in the CNS may turnout troublesome as the better alternative at this time is through viral vectors such as adeno-associated virus (AAV). Whether the faith of CRISPR/Cas9 as a restorative tool to tackle AD will be fulfilled, remains to be witnessed, further more studies is inevitable to emend the procedure and to evince proven potency in animal models of AD.
References
- Jones EL, Kalaria RN, Sharp SI, O’Brien JT, Francis PT, et al. Genetic associations of autopsy-confirmed vascular dementia subtypes. Dement GeriatrCognDisord. 2011; 31: 247–253. Ref.: https://goo.gl/VrBuco
- Mirra SS, Heyman A, McKeel D, Sumi SM, Crain BJ, et al. The consortium to establish a registry for Alzheimer’s disease (CERAD) part II. Standardization of the neuropathological assessment of Alzheimer’s disease. Neurol. 1991; 41: 479–486. Ref.: https://goo.gl/GZ4PKL
- Terry RD, Masliah E, Salmon DP, Butters N, DeTeresa R, et al. Physical basis of cognitive alterations in Alzheimer’s disease: synapse loss is the major correlate of cognitive impairment. Ann Neurol. 1991; 30: 572–580. Ref.: https://goo.gl/UBTCiK
- DeKosky ST, Scheff SW, Styren SD. Structural correlates of cognition in dementia: Quantification and assessment of synapse change. Neurodegeneration. 1996; 5: 417–421. Ref.: https://goo.gl/vxUsux
- Coleman PD, Yao PJ. Synaptic slaughter in Alzheimer’s disease. Neurobiol Aging. 2003; 24: 1023–1027. Ref.: https://goo.gl/nezMzY
- Lacor PN, Buniel MC, Chang L, Fernandez SJ, Gong Y, et al. Synaptic targeting by Alzheimer’s-related amyloid beta oligomers. J Neurosci. 2004; 24: 10191–10200. Ref.: https://goo.gl/st1b1F
- Lambert MP, Barlow AK, Chromy BA, Edwards C, Freed R, et al. Diffusible, nonfibrillar ligands derived from Abeta1-42 are potent central nervous system neurotoxins. Proc Natl Acad Sci U S A. 1998; 95: 6448–6453. Ref.: https://goo.gl/wyxW1e
- McLean CA, Cherny RA, Fraser FW, Fuller SJ, Smith MJ, et al. Soluble pool of Abeta amyloid as a determinant of severity of neurodegeneration in Alzheimer’s disease. Ann Neurol. 1999; 46: 860–866. Ref.: https://goo.gl/ETDLih
- Broersen K, Rousseau F, Schymkowitz J. The culprit behind amyloid beta peptide related neurotoxicity in Alzheimer’s disease: Oligomer size or conformation? Alzheimers Res Ther. 2010; 2: 12. Ref.: https://goo.gl/sSVGiS
- Rohn TT, Kim N, Isho NF, Mack JM. The Potential of CRISPR/Cas9 Gene Editing as a Treatment Strategy for Alzheimer’s Disease. J Alzheimers Dis Parkinsonism. 2018; 8: 439. Ref.: https://goo.gl/7NDf8r
- Mojica FJ, Diez-Villasenor C, Garcia-Martinez J, Soria E. Intervening sequences of regularly spaced prokaryotic repeats derive from foreign genetic elements. J MolEvol. 2005; 60: 174–182. Ref.: https://goo.gl/oKLqZy
- Pourcel C, Salvignol G, Vergnaud G. CRISPR elements in Yersinia pestis acquire new repeats by preferential uptake of bacteriophage DNA, and provide additional tools for evolutionary studies. Microbiology. 2005; 151: 653–663. Ref.: https://goo.gl/wpw3tY
- Barrangou R, Fremaux C, Deveau H, Richards M, Boyaval P, et al. CRISPR provides acquired resistance against viruses in prokaryotes. Science. 2007; 315: 1709–1712. Ref.: https://goo.gl/jRYzbU
- Bolotin A, Quinquis B, Sorokin A, Ehrlich SD. Clustered regularly interspaced short palindrome repeats (CRISPRs) have spacers of extrachromosomal origin. Microbiology. 2005; 151: 2551–2561. Ref.: https://goo.gl/j9tdyj
- Deltcheva E, Chylinski K, Sharma CM, Gonzales K, Chao Y, et al. CRISPR RNA maturation by trans-encoded small RNA and host factor RNase III. Nature. 2011; 471: 602–607. Ref.: https://goo.gl/LgTULX
- Saudou F, Humbert S. The biology of huntingtin. Neuron. 2016; 89: 910–926. Ref.: https://goo.gl/WGjZZv
- Bates GP, Dorsey R, Gusella JF, Hayden MR, Kay C, et al. Huntington disease. Nat Rev Dis Primers. 2015; 1: 15005. Ref.: https://goo.gl/sYTJU7
- Dabrowska M, Juzwa W, Krzyzosiak WJ, Olejniczak M. Precise excision of the CAG tract from the huntingtin gene by cas9 nickases. Front Neurosci. 2018; 12: 75. Ref.: https://goo.gl/juHxQe
- Schellenberg GD, Bird TD, Wijsman EM, Orr HT, Anderson L, et al. Genetic linkage evidence for a familial Alzheimer’s disease locus on chromosome 14. Science. 1992; 258: 668–671. Ref.: https://goo.gl/q2EmRY
- Levy-Lahad E, Wijsman EM, Nemens E, Anderson L, Goddard KA, et al. A familial Alzheimer’s disease locus on chromosome 1. Science. 1995; 269: 970–973. Ref.: https://goo.gl/2SYDSQ
- Vetrivel KS, Zhang YW, Xu H, Thinakaran G. Pathological and physiological functions of presenilins. MolNeurodegener. 2006; 1: 4. Ref.: https://goo.gl/Dbk6wU
- Pires C, Schmid B, Petræus C, Poon A, Nimsanor N, et al. Generation of a gene-corrected isogenic control cell line from an Alzheimer’s disease patient iPSC line carrying a A79V mutation in PSEN1. Stem Cell Res. 2016; 17: 285–288. Ref.: https://goo.gl/Bns14i
- Poon A, Schmid B, Pires C, Nielsen TT, Hjermind LE, et al. Generation of a gene-corrected isogenic control hiPSC line derived from a familial Alzheimer’s disease patient carrying a L150P mutation in presenilin 1. Stem Cell Res. 2016; 17: 466–469. Ref.: https://goo.gl/TAsC6J
- György B, Lööv C, Zaborowski M, Takeda S, Kleinstiver B, et al. CRISPR/Cas9 mediated disruption of the swedish APP allele as a therapeutic approach for early-onset alzheimer’s disease. MolTher Nucleic Acids. 2018; 11: 429–440. Ref.: https://goo.gl/5Q2R3R
- Sun J, Carlson-Stevermer J, Das U, Shen M, Delenclos M, et al. A CRISPR/Cas9 based strategy to manipulate the Alzheimer’s amyloid pathway. 2018 bioRxiv. Ref.: https://goo.gl/3NdTq9
- Das U, Scott DA, Ganguly A, Koo EH, Tang Y, et al. Activity-induced convergence of APP and BACE-1 in acidic microdomains via an endocytosis-dependent pathway. Neuron. 2013; 79: 447–460. Ref.: https://goo.gl/Q3nKnq
- Eisenstein M. Genetics: Finding risk factors. Nature. 2011; 475: S20–22. Ref.: https://goo.gl/GNjWRL
- Weisgraber KH, Rall SC, Jr, Mahley RW. Human E apoprotein heterogeneity. Cysteine-arginine interchanges in the amino acid sequence of the apo-E isoforms. J Biol Chem. 1981; 256: 9077–9083. Ref.: https://goo.gl/dn7yof
- Farrer LA, Cupples LA, Haines JL, Hyman B, Kukull WA, et al. Effects of age, sex, and ethnicity on the association between apolipoprotein E genotype and Alzheimer disease. A meta-analysis. APOE and alzheimer disease meta-analysis consortium. JAMA. 1997; 278: 1349–1356. Ref.: https://goo.gl/VTAWzn
- Saunders AM, Strittmatter WJ, Schmechel D, George-Hyslop PH, Pericak-Vance MA, et al. Association of apolipoprotein E allele epsilon 4 with late-onset familial and sporadic Alzheimer’s disease. Neurol. 1993; 43: 1467–1472. Ref.: https://goo.gl/nTC61T
- Kanekiyo T, Xu H, Bu G. ApoE and Aβ in Alzheimer’s disease: accidental encounters or partners? Neuron. 2014; 81: 740–754. Ref.: https://goo.gl/vuR6oA
- Wang C, Najm R, Xu Q, Jeong DE, Walker D, et al. Gain of toxic apolipoprotein E4 effects in human iPSC-derived neurons is ameliorated by a small-molecule structure corrector. Nat Med. 2018; 24: 647–657. Ref.: https://goo.gl/rvcjWZ
- Dong LM, Weisgraber KH. Human apolipoprotein E4 domain interaction. Arginine 61 and glutamic acid 255 interact to direct the preference for very low density lipoproteins. J Biol Chem. 1996; 271: 19053–19057. Ref.: https://goo.gl/bff8mX
- Prince M, Comas-Herrera A, Knapp M, Guerchet M, Karagiannidou M. Coverage, quality and costs now and in the future. Alzheimer’s Disease International; London: 2016. World Alzheimer Report 2016. Improving healthcare for people living with dementia.